Back to Journals » Clinical Interventions in Aging » Volume 18

Sarcopenia and COVID-19 Outcomes
Authors Wang Y, Tan S, Yan Q, Gao Y
Received 19 November 2022
Accepted for publication 2 March 2023
Published 9 March 2023 Volume 2023:18 Pages 359—373
DOI https://doi.org/10.2147/CIA.S398386
Checked for plagiarism Yes
Review by Single anonymous peer review
Peer reviewer comments 4
Editor who approved publication: Prof. Dr. Nandu Goswami
Yuhan Wang, Shuwen Tan, Qihui Yan, Ying Gao
Department of Endocrinology and Metabolism, The First Hospital of Jilin University, Changchun, People’s Republic of China
Correspondence: Ying Gao, Department of Endocrinology and Metabolism, The First Hospital of Jilin University, No. 1 Xinmin Street, Changchun, Jilin, 130021, People’s Republic of China, Tel +86-15804300435, Email [email protected]
Abstract: Coronavirus disease 2019 (COVID-19) spread rapidly and became a severe global public health threat. Older adults have a high risk of COVID-19 and its associated mortality. Sarcopenia has emerged as a predictor of poor outcomes in COVID-19 patients, including lengthy hospital stays, mortality, intensive care unit admission, need for invasive mechanical ventilation, and poor rehabilitation outcomes. Chronic inflammation, immune dysfunction, respiratory muscle dysfunction, and swallowing dysfunction may underlie the association between sarcopenia and the poor outcomes of COVID-19 patients. Interleukin 6 receptor blockers (tocilizumab or sarilumab) are recommended for treating patients with severe COVID-19, and their therapeutic effects on sarcopenia are of great interest. This review aimed to analyze the current reports on the association between sarcopenia and COVID-19 and provide an update on the contribution of sarcopenia to the severity and adverse outcomes of COVID-19 and its underlying mechanisms. We also aimed to explore the different screening tools for sarcopenia concurrent with COVID-19, and advocate for early diagnosis and treatment of sarcopenia. Given that the fight against the COVID-19 pandemic may be long-term, further research into understanding the effects of sarcopenia in patients infected with the Omicron variant is necessary.
Keywords: COVID-19, sarcopenia, skeletal muscle, inflammation, IL-6
Introduction
Coronavirus disease 2019 (COVID-19) spread rapidly and became a severe global public health threat.1 As of February 28, 2023, COVID-19 had been confirmed in 758,390,564 persons globally and had accounted for 6,859,093 deaths.2 The COVID-19 pandemic has heavily burdened health-care systems and the economies of most countries.3,4 The rapid spread of COVID-19 is currently relatively under control; however, several countries are still battling with waves of COVID-19.5,6 The majority of patients with COVID-19 have mild to moderate symptoms; however, 10–20% develop severe conditions and require hospitalization and intensive care treatment. The fatality rate is higher for older people and is reported to be approximately 20% for patients aged ≥80 years.7 The established risk factors for worse prognoses of COVID-19 are old age, male sex, obesity, and comorbidities such as chronic lung diseases, diabetes, and cardiovascular diseases.8–11
Sarcopenia is defined as low muscle mass with low muscle function (grip strength or physical performance)12 and was formally recognized as a disease entity with the assignment of an International Classification of Diseases, Tenth Revision, Clinical Modification code (M62.84) in 2016.13 The definitions by the European Working Group on Sarcopenia in Older People 2 (2019), Asian Working Group for Sarcopenia (2019), International Working Group on Sarcopenia, and Foundation for the National Institute of Health are the most used. All definitions include at least two parameters, but the use of different cutoff values leads to a lack of standardization in clinical practice. Several techniques including CT, MRI, dual-energy X-ray absorptiometry, and bioimpedance analysis (BIA) are used to estimate muscle mass; dual-energy X-ray absorptiometry and BIA are recommended in clinical practice. Muscle strength, which is usually measured as grip strength, is a well-validated parameter for determining sarcopenia. The measures of physical performance include the gait speed test, 400-m timed walk, the Short Physical Performance Battery, and the Timed Up and Go test.16 Sarcopenia is commonly considered an age-related process in older adults, with a prevalence of 10–27% for older adults aged ≥60 years14 and 41% for older nursing home residents.15 Sarcopenia may also be secondary to diseases, malnutrition, and physical inactivity.12,16
Previous studies have demonstrated that sarcopenia increases vulnerability to adverse outcomes, such as poorer quality of life, falls, and fractures, in older adults;17 increased hospitalization and mortality rates in patients with chronic diseases;18,19 and poorer overall survival of patients with cancer.20 Sarcopenia is predictive of a higher risk of community-acquired pneumonia in older adults,21 as well as 90-day mortality in patients with aspiration pneumonia.22 In addition, sarcopenia is associated with unfavorable outcomes in critically ill patients, including a greater likelihood of the use of invasive mechanical ventilation (IMV), difficult weaning, and mortality.23–25
Currently, increased attention has been paid to sarcopenia in patients with COVID-19 due to its potential effects. However, the associations between sarcopenia and COVID-19 outcomes have not been fully elucidated. This review aimed to provide an update on the contribution of sarcopenia to the severity and adverse outcomes of COVID-19 and its potential mechanism. We explored the available screening tools for sarcopenia concurrent with COVID-19 and advocate for its early diagnosis and management.
Clinical Evidence Linking Sarcopenia to Poor Outcomes in Patients with COVID-19
Methods
The PubMed database was searched for articles published from the beginning of publication on COVID-19 until September 2022. The following search terms were used: “sarcopenia”, “low skeletal muscle”, “muscle mass”, “grip strength”, “muscle quality”, “intramuscular adipose tissue”, “myosteatosis” and “COVID-19”. The search was restricted to human studies, and the language was limited to English. We included publications reporting data on the association between sarcopenia and poor outcomes in patients with COVID-19. We screened out non-relevant articles by reviewing the titles and abstracts. Subsequently, the full texts of the remaining articles were analyzed to determine the final inclusion. We retrieved 266 articles manually, of which 19 were the most relevant (Table 1).
![]() |
Table 1 Summary of the Association Between SARC-F, Grip Strength, Muscle Mass or Quality, and Outcomes in Patients with COVID-19 |
Results
The included studies investigated the associations between strength, assistance in walking, rising from a chair, climbing stairs, falls (SARC-F) score, grip strength, muscle mass or quality, and poor outcomes in patients with COVID-19. Various tools, such as BIA, CT, and ultrasonography, have been used to assess muscle mass and/or quality among COVID-19 patients in the literature (Table 1).
SARC-F
SARC-F is a 5-item self-reported questionnaire for assessing strength, walking assistance, rising from a chair, climbing stairs, and falls.26 It has a low sensitivity but high specificity and can be a convenient and inexpensive tool for sarcopenia risk screening in clinical practice.12,27,28 In the same vein, SARC-F may be a simple and feasible tool for early detection of the high risk of sarcopenia in patients with COVID-19. In two studies,29,30 a total score of ≥4 was indicative of a high risk of sarcopenia in patients with COVID-19. A prospective cohort study of 114 patients hospitalized for COVID-19 (age ≥60 years) from China showed that a higher sarcopenia risk was associated with more severe COVID-19.30 In a cross-sectional study involving 337 consecutively hospitalized patients with COVID-19 (age ≥65 years), a higher risk of sarcopenia was associated with a high risk of mortality.29
Grip Strength
Muscle strength is among the main indicators of muscle function in sarcopenia.12 Grip strength is recommended as a good measure of muscle strength and can be measured using a hand dynamometer.31 Being simple and inexpensive, the measurement of grip strength in patients with COVID-19 is noteworthy.32 Kara et al33 and Tuzun et al34 reported that low grip strength was a predictor of the disease severity in hospitalized patients with COVID-19. A study by Gil et al showed that increased grip strength was associated with a shorter hospital stay for patients with moderate to severe COVID-19.35 In a retrospective study from Italy, grip strength was a key predictor of the rehabilitation outcomes of post-acute COVID-19 patients.36
BIA
BIA is recommended as an affordable, easy-to-use, and portable measurement of muscle mass in routine clinical practice.12,37 BIA does not directly measure muscle mass, but it estimates muscle mass by sending a low electrical current into the body.12 In a retrospective study from Italy, skeletal muscle mass measured by BIA appeared to be a key predictor of rehabilitation outcomes in patients with post-acute COVID-19.36 However, the reliability of BIA may reduce when patients have abnormal hydration.38 Additionally, it may not be suitable for evaluating critically ill patients in the ICU; these patients are usually edematous due to fluid overload, and muscle mass can be overestimated.39
CT
CT is considered the gold standard for non-invasive measurements of muscle mass and is routinely performed with no additional radiation burden for patients with COVID-19. Conventionally, cutoffs for skeletal muscle measurements are evaluated at the level of the third lumbar (L3) vertebra on abdominal CT, because skeletal muscle mass in this region correlates well with total body skeletal muscle mass. The skeletal muscle mass index (SMI) was calculated as the division of the skeletal muscle area by the square of the height. A study by McGovern et al showed that low SMI at the level of the L3 vertebra was associated with a higher 30-day mortality rate among patients with COVID-19.40 In patients with COVID-19 admitted to the ICU, low SMI at the L3 level was associated with a high likelihood of tracheostomy, prolonged ICU stays, and longer durations of hospital stay;41 Damanti et al also showed that low muscle mass at the L1, L2, or L3 level was associated with extubation failure, prolonged ICU stay, and higher hospital mortality rate.42
Chest CT has been used to assess the presence and severity of pneumonia in patients with COVID-19, and it can also be used to identify patients with lower muscle mass who have a high risk of worse outcomes; it is, therefore, useful for both diagnostic and prognostic assessments. Skeletal muscle mass at the level of the twelfth thoracic vertebra (T12) on chest CT is significantly associated with the skeletal muscle mass at L3.43,44 A study of 121 patients with COVID-19 from South Korea showed that lower SMI at the T12 level on chest CT was independently associated with prolonged hospital stay but not mortality.45 In a retrospective study conducted in Italy, lower muscle mass at the T5 or T12 level on chest CT was independently associated with ICU admission and increased hospital mortality among patients with COVID-19.46 Giraudo et al also showed that reduced muscle mass at the T12 level was a predictor of ICU hospitalization of patients with COVID-19.47 However, in a retrospective cohort study involving 519 hospitalized patients with COVID-19 from Mexico, the SMI on CT at the T12 level was not associated with mortality, ICU admission, or IMV need.48 The pectoralis muscle area is calculated as the sum of the bilateral pectoralis major and minor muscle areas. Ufuk et al reported that the pectoralis muscle area and pectoralis muscle index on chest CT were associated with prolonged hospital stays, intubation, and death of adult patients with COVID-19.49
CT has also been used to evaluate muscle quality using fat infiltration into the muscle and attenuation of the skeletal muscle.12 Muscle quality refers to changes in muscle architecture and composition, as well as muscle function delivered per unit of muscle mass.12 When the capacity of subcutaneous and visceral tissue for fat storage is exceeded, lipids can be stored within the skeletal muscle as intramuscular adipose tissue (IMAT).50 Viddeleer et al showed that a larger cross-sectional area of IMAT at the T12 level on chest CT was a risk factor for poor survival among patients with COVID-19.51 Yang et al found that high IMAT at the L3 level on abdominal CT was associated with disease severity and increased need for mechanical ventilation and risk of death.52 Higher paraspinal muscle radiodensity is a proxy measure of muscle fat deposition. Feng et al reported that higher paraspinal muscle radiodensity at the T12 level on chest CT was associated with a reduced risk of disease aggravation and a decreased likelihood of prolonged viral shedding in female patients with COVID-19 admitted to the ICU.53 Skeletal muscle radiation attenuation may be a reflection of myosteatosis. In a retrospective study conducted in China, lower skeletal muscle radiation attenuation at the T12 level on chest CT was associated with an increased risk of progression to severe illness in patients with COVID-19.54
Ultrasonography
Ultrasonography may be an innovative tool for assessing muscle mass and quality in patients with COVID-19.12 It is valid and reliable and can be performed at the bedside by trained clinicians.12 It ensures longitudinal monitoring of muscle loss, reduces the risks of contamination associated with mobilization to the radiology unit, and reduces radiation exposure of patients. Gil et al reported that low muscle mass assessed in the vastus lateralis cross-sectional area on B-mode ultrasound was predictive of prolonged hospital stay of patients with moderate to severe COVID-19.35 Muscle indices derived from the psoas muscle were also predictors of poor outcomes. A study by Kremer et al showed that the low psoas muscle area index was associated with a higher 30-day mortality rate of patients with COVID-19.55 The clinical studies on the relationships between muscle mass and quality assessed by ultrasonography and the adverse outcomes of COVID-19 are limited, and further large-scale prospective studies are needed to establish the prognostic roles of muscle mass and quality assessed by ultrasonography in patients with COVID-19.
In summary, these data suggest that sarcopenia is an important risk factor for disease exacerbation and poor outcomes in patients with COVID-19. Therefore, patients with COVID-19 should be routinely screened for sarcopenia in clinical practice using available methods. Early diagnosis of sarcopenia appears to be of marked importance for identifying patients with COVID-19 at risk of unfavorable outcomes. However, different screening tools and diagnostic cut-off points for sarcopenia have been used in the above studies. Further large-scale prospective studies for exploring the relationships between sarcopenia and COVID-19 outcomes are warranted.
Potential Mechanisms Underlying the Relationship Between Sarcopenia and COVID-19 Poor Outcomes
Sarcopenia has emerged as a predictor of poor outcomes in patients with COVID-19. Chronic inflammation, immune dysfunction, respiratory muscle dysfunction, and swallowing dysfunction may underlie the association between sarcopenia and the poor outcomes of COVID-19 (Figure 1).
Chronic Inflammatory State
“Inflammaging” is characterized as an age-related, chronic, low-grade inflammatory state with slightly elevated plasma concentrations of pro-inflammatory factors, such as interleukin 6 (IL-6), tumor necrosis factor-α (TNF-α), and C-reactive protein, contributing to the pathogenesis of age-related sarcopenia.56,57 Chronic low-grade inflammation affects both muscle protein anabolism and catabolism through direct or indirect mechanisms.57 Higher IL-6 and TNF-α serum concentrations were observed in sarcopenic older adults than in their non-sarcopenic counterparts.58 Elevated concentrations of TNF-α were associated with an increased risk of sarcopenia in older people.59 A prospective cohort study showed that high concentrations of IL-6 and C-reactive protein were associated with the loss of total appendicular skeletal muscle mass in non-sarcopenic older adults.60 A meta-analysis also showed that elevated concentrations of circulating inflammatory markers were significantly correlated with lower skeletal muscle mass and muscle strength.61 Taken together, skeletal muscle may be a potential central integrator between inflammation and sarcopenia during the biological process of aging.62
Cytokine storm is an uncontrolled systemic inflammatory condition characterized by high production of pro-inflammatory cytokines and chemokines, including IL-6, IL-8, TNF-α, and interferon, resulting from an overreaction of the immune system.63 Cytokine storm may rapidly lead to acute respiratory distress syndrome, multiple organ failure, and death in patients with severe COVID-19.64 Previous studies have reported significantly higher serum concentrations of proinflammatory cytokines in the majority of patients with severe COVID-19, suggesting that cytokine storms are associated with disease severity.65,66 A low-grade chronic inflammatory state may be associated with an increased risk of a cytokine storm in patients with severe COVID-19.67 Therefore, sarcopenic patients with COVID-19 may be more likely to develop unfavorable outcomes because of a low-grade chronic inflammatory state.
Immune Dysfunction
Skeletal muscle is currently also regarded as a secretory organ that can produce and release various soluble factors referred to as “myokines”, in addition to being a locomotor unit.68,69 Several of these myokines are secreted when the skeletal muscle contracts. Myokines can induce muscle regeneration, regulate whole-body homeostasis, and maintain immune function.68 Therefore, skeletal muscle serves as a potent regulator of immune function. A key mechanism underlying impaired immunity in sarcopenic individuals is the abnormal expression of myokines, such as IL-15 and IL-6.70
IL-15 is highly expressed in skeletal muscle71 and declines in aging rats.72 In animal and in vitro studies, IL-15 has been demonstrated to have positive effects on skeletal muscle. IL-15 has been considered an anabolic factor in muscle protein metabolism,73 and it promotes human myotube development and mitigates the deleterious effects of TNF-α on myogenesis.74 Beneficial metabolic effects of IL-15 on skeletal muscle have been reported.75–78 IL-15 increases glucose uptake through the Janus kinase 3/signal transducers and activators of transcription 3 pathway75 or the Adenosine monophosphate (AMP)-activated protein kinase pathway,76 regulates lipid oxidation via peroxisome proliferation activated receptor δ,77 and mediates mitochondrial activity through the induction of peroxisome proliferation activated receptor δ78 in the skeletal muscle. The role of IL-15 in muscle physiology suggests that it may be used as a potential sarcopenia biomarker.62 A cross-sectional study showed that lower plasma IL-15 concentrations were associated with sarcopenia in older adults.79 However, further prospective studies are necessary to determine the association between decreased IL-15 concentrations and reduced muscle mass and strength.
IL-15 is characterized as a critical immunomodulator with anti-viral properties.80 NK and CD8 T cells are required for effective viral clearance. These cells should be activated, and they should migrate to sites of infection and survive and proliferate, and IL-15 can regulate each of these processes.80 In IL-15-deficient mice, defects in CD8 T and NK cells and compromised host defense responses to viral pathogens are observed,81 indicating that a lack of IL-15 signaling may lead to poor immune responses against COVID-19. Thus, we speculate that the interaction between skeletal muscle and the immune system may justify the association between sarcopenia and the poor outcomes of patients with COVID-19. Reductions in IL-15 concentrations in patients with sarcopenia may affect the host defense system against COVID-19.
Respiratory Muscle Dysfunction
The diaphragm is the main inspiratory muscle. Greising et al demonstrated that diaphragmatic sarcopenia was represented by reductions in diaphragm force and fiber size (diaphragm weakness and fiber atrophy) in aging rats.82 In the diaphragm, sarcopenia affects the cross-sectional areas of type IIx and/or IIb muscle fibers.83,84 The functional impact of diaphragmatic sarcopenia is a reduction in the maximum transdiaphragmatic pressure, resulting in an impaired ability to perform expulsive, high-force non-ventilatory behaviors (coughing and sneezing).85 The performance of expulsive and non-ventilatory activities is critical for airway clearance. Therefore, diaphragmatic sarcopenia may increase the risk of developing pneumonia and other respiratory system infections.86
Transdiaphragmatic pressure is the gold standard measurement of diaphragm function. However, measuring transdiaphragmatic pressure requires nasogastric catheterization, considerable expertise, and patient cooperation, which make it impossible for critically ill patients.87,89 Alternatively, diaphragm function can be noninvasively evaluated using ultrasound. Diaphragm thickness, diaphragm thickening fraction, and diaphragmatic excursion measured by ultrasound are putative estimators of diaphragm function.88 Sonographic measurements of diaphragm thickness provide a useful tool for assessing and monitoring for diaphragm atrophy and inactivity during mechanical ventilation.89 Diaphragm thickening fraction may guide clinicians in assessing diaphragm function.90 Despite its extensive use in the ICU,91,92 few studies have reported on the relationship between transdiaphragmatic pressure and diaphragm thickening fraction,89,93 and further investigations are required. Corradi et al reported that low diaphragmatic thickening fraction was a predictor of continuous positive airway pressure failure and the need for invasive ventilation in critically ill patients with COVID-19.94 The same author reported that reduced diaphragm thickness measured by ultrasonography increased the invasive mechanical ventilation need or risk of death of hospitalized patients with COVID-19.95 The observed association between reduced diaphragm thickness and poor clinical outcomes may be attributed to the limitation of the inspiratory pump strength, which promotes the progression from mild to severe respiratory failure, necessitating the need for IMV for cases of worsening pneumonia where the respiratory system becomes less compliant.95 In summary, we speculate that respiratory muscle dysfunction induced by diaphragmatic sarcopenia increases the chances of contraction and the risk of poor outcomes in patients with COVID-19. Future studies are needed to further elucidate the relationships between respiratory muscle dysfunction and COVID-19 outcomes.
Swallowing Dysfunction
Dysphagia is characterized by difficulty swallowing96 and is a common problem in frail older adults.97 “Sarcopenic dysphagia” refers to a swallowing disorder caused by sarcopenia of the entire body and swallowing muscles.98,99 A study by Campo et al showed that sarcopenic dysphagia was associated with increased mortality rates among hospitalized older adults.100 An international expert panel on dysphagia proposed that several patients with COVID-19 may have pre-existing dysphagia. In older hospitalized patients with COVID-19, sarcopenia may also contribute to dysphagia symptoms.101 Sarcopenic dysphagia has attracted increasing interest in recent years; however, the association between pre-existing sarcopenic dysphagia and poor prognosis in patients with COVID-19 has not been thoroughly explored. Additionally, it has been estimated that approximately 30% of critically ill patients with COVID-19 experience post-extubation dysphagia,102,103 which is associated with higher disease severity scores and longer duration of mechanical ventilation.103 Thus, we speculate that pre-existing sarcopenic dysphagia may be associated with poor outcomes in patients with COVID-19. Future studies are required to further elucidate the relationships between pre-existing sarcopenic dysphagia and COVID-19 outcomes.
Nutritional Supplementation and Physical Exercise of Sarcopenia in COVID-19 Patients
No specific drugs have been approved for sarcopenia. However, nutritional therapy and physical exercise are the mainstays of sarcopenia treatment in patients with COVID-19. Protein supplements combined with physical exercise may be effective for treating sarcopenia and COVID-19. Further prospective studies exploring whether these and other interventions can improve the outcomes of patients with COVID-19 are needed.
Nutritional Supplementation
The ingestion of dietary protein is necessary for muscle protein synthesis.104 Protein supplementation increased skeletal muscle mass during 24 weeks of resistance-type exercise training in a study.105 Dietary protein supplementation also improved physical performance in frail older adults in another study.106 The International Conference on Sarcopenia and Frailty Research recommends protein supplementation or a protein-rich diet for older adults with sarcopenia.107 According to the recommendation of the Society of Sarcopenia, Cachexia, and Wasting Disorders, protein intake of 1–1.5 g/kg/day combined with physical exercise appears to be reasonable for patients with sarcopenia.108 In patients with injury or severe illness, or evidence of a catabolic/pro-inflammatory state, protein doses of up to 2 g/kg/day may be appropriate.108 A high protein diet may be beneficial for patients with COVID-19, as proteins may prevent further muscle protein breakdown for gluconeogenesis and protect patients from progressing to a further catabolic state.109 Patients with COVID-19 admitted to the ICU often have higher protein requirements, and nutritional recommendations may vary between 1.2 and 2.5 g/kg/day of protein.110,111
Vitamin D is essential for the maintenance of skeletal muscle health. There is accumulating evidence that vitamin D may play an important role in muscle regeneration and damage.112 Although a meta-analysis of twenty-nine studies revealed that vitamin D supplementation improved muscle strength in individuals with low vitamin D concentrations,113 there is insufficient evidence to recommend vitamin D supplementation for sarcopenia according to the International Conference on Sarcopenia and Frailty Research and Society of Sarcopenia, Cachexia, and Wasting Disorders.107,108 Vitamin D may play a role in modulating the immune response to severe acute respiratory syndrome coronavirus 2 (SARS-CoV-2).114 A meta-analysis showed that vitamin D deficiency increased susceptibility to COVID-19 and the risk of developing severe COVID-19.115 Several randomized controlled trials have reported that a high dose of vitamin D may have benefits for the treatment of COVID-19, including reducing inflammatory markers116 and the need for ICU treatment.117 However, a randomized controlled trial by Murai et al reported that a single high dose of vitamin D did not significantly reduce the length of hospital stay for patients with COVID-19.114 The National Institute for Health and Care Excellence has recommended that there is limited evidence to support taking vitamin D supplements to prevent or treat COVID-19.118 Further randomized control trials evaluating the administration of high doses of vitamin D for COVID-19 are needed.
Physical Exercise
Evidence-based clinical practice guidelines have recommended physical exercise as a treatment for sarcopenia.107 Evidence from studies shows that resistance exercise improves muscle mass, muscle strength, and physical performance in patients with sarcopenia.105,119,120 Physical exercises strengthen the immune system and may provide benefits against viral communicable diseases. Active mobilization interventions are recommended for hospitalized patients with COVID-19, including bed mobility activities and active range of motion exercises.121 Notably, physical exercise should be introduced gradually to patients with severe COVID-19, particularly those requiring intensive care. Exercises with assistants or auxiliary appliances should begin at low intensity and gradually increase to appropriate intensities under the supervision of physicians.122 Regular exercise of adequate intensity is considered auxiliary for improving the host immune response against COVID-19.123
Are IL-6 Receptor Blockers Promising for the Treatment of Sarcopenia in Severe COVID-19 Patients?
Elevated concentrations of pro-inflammatory cytokines (notably IL-6, IL-18, interferon, and TNF-α) have been commonly reported for patients with severe COVID-19. These cytokines are often considered part of a dysregulated host immune response to SARS-CoV-2 that worsens disease severity.124 IL-6 receptor blockers engage the membrane-bound and soluble IL-6 receptor,125 blocking cytokine activation and regulating the host immune response to SARS-CoV-2.124 Tocilizumab and sarilumab, as IL-6 receptor blockers, have been approved for the treatment of rheumatoid arthritis.126 A network meta-analysis including 30 randomized clinical trials with 10,618 patients hospitalized for COVID-19 showed that the administration of IL-6 receptor blockers, compared with placebo or usual care, was associated with lower 28-day all-cause mortality rates and delayed progression to the need for invasive mechanical ventilation or death, cardiovascular support or death, and kidney replacement therapy or death of patients not receiving support for the corresponding organ at randomization.127 Currently, the World Health Organization strongly recommends tocilizumab or sarilumab for treating patients with severe COVID-19.128
Prolonged exposure to IL-6 promotes muscle atrophy and wasting by disrupting muscle anabolism and energy homeostasis.129 The concomitant activities of other pro-inflammatory cytokines, such as TNF-α, may create the physiological environment, leading to muscle catabolism.70 However, in the absence of proinflammatory cytokines, exercise-induced pulsatile IL-6 stimulates anabolic pathways associated with muscle growth and myogenesis.129 A recent review concluded that for sarcopenia, IL-6 signaling may shift towards the pro-inflammatory profile as skeletal muscle function declines with age.70 These findings suggest that IL-6 receptor blockers may have the potential to treat sarcopenia. Several preclinical studies have reported that IL-6 receptor blockers attenuate muscular atrophy and facilitated muscle regeneration.130,131 In IL-6 transgenic mice, treatment with anti-mouse IL-6 receptor antibody blocked the activation of proteolytic systems associated with muscle atrophy in the gastrocnemius muscles.130 Additionally, the acceleration of muscle regeneration was observed on day 7 after the anti-mouse IL-6 receptor antibody treatment of mice with cardiotoxin-induced muscle damage. The anti-mouse IL-6 receptor antibody, which was primarily found in infiltrated macrophages in the damaged muscle may facilitate muscle regeneration through immune modulation.131 However, the administration of a monoclonal antibody against the IL-6 receptor in mice for five weeks led to increased muscle inflammation, with no significant change in muscle regeneration and muscle function.132 A prospective cohort study showed that IL-6 receptor blockers may be effective for treating sarcopenia with concomitant rheumatoid arthritis. A significant increase in the appendicular lean mass was observed after treatment with tocilizumab (an IL-6 receptor blocker) for one year. However, data on muscle strength and function were not provided by this study.133 Therefore, the effects of IL-6 receptor blockers on skeletal muscle remain controversial, and whether targeting IL-6 will be a useful therapeutic strategy to treat sarcopenia in patients with severe COVID-19 has not been established. The administration of IL-6 receptor blockers (tocilizumab or sarilumab) is promising for patients with severe or critical COVID-19. Future studies on the roles of IL-6 in skeletal muscle will provide a new basis for therapeutic approaches to treat sarcopenia in patients with severe COVID-19.
Conclusion
The COVID-19 outbreak presented significant challenges in communities and for health-care systems globally. The established risk factors for worse prognoses of COVID-19 patients are older age, male sex, obesity, and comorbidities including chronic lung diseases, diabetes, and cardiovascular diseases. Sarcopenia may be an emerging risk factor. The potential mechanisms underlying the association between sarcopenia and poor COVID-19 outcomes are chronic inflammatory state, immune dysfunction, respiratory muscle dysfunction, and swallowing dysfunction. Previous studies have indicated that sarcopenia is associated with poor prognoses such as prolonged hospital stay, mortality, ICU admission, or IMV need in COVID-19 patients. Therefore, sarcopenia should be routinely screened in clinical practice using available methods for these patients. In the meantime, the prevention and treatment of sarcopenia may prevent the worsening of COVID-19. Treatments should be holistic and focused on both physical exercise and nutritional interventions. The administration of IL-6 receptor blockers (tocilizumab or sarilumab) is promising for patients with severe COVID-19. However, reports on the effects of IL-6 receptor blockers on the skeletal muscle are controversial, and whether targeting IL-6 will be a useful therapeutic strategy for treating sarcopenia in patients with severe COVID-19 is not known. Omicron is becoming the dominant strain in most countries,134,135 and it seems to be less pathogenic than the earlier SARS-CoV-2 strains and four other variants of concern.134 With the emergence of SARS-CoV-2 variants, the association between sarcopenia and COVID-19 may also have changed. The association of sarcopenia with poor outcomes in patients infected with the Omicron variant has not yet been studied. Given that the fight against the COVID-19 pandemic may be long-term, further research into understanding the effects of sarcopenia in patients infected with the Omicron variant is necessary.
Acknowledgments
This study was funded by Finance Department of Jilin Province (JLSCZD2019-061). The authors express thanks to Figdraw for providing the pictures of immune cells and human body.
Disclosure
The authors report no conflicts of interest in this work.
References
1. Tarik Jasarevic CL, Chaib F. Statement on the second meeting of the International Health Regulations (2005) Emergency Committee regarding the outbreak of novel coronavirus (2019-nCoV); 2020. Available from: https://www.who.int/news/item/30-01-2020-statement-on-The-second-meeting-of-The-international-health-regulations-(2005)-emergency-committee-regarding-The-outbreak-of-novel-coronavirus-(2019-ncov).
2. World Health Organization. WHO coronavirus (COVID-19) dashboard; 2022. Available from: https://covid19.who.int/.
3. Else H. The pandemic’s true health cost: how much of our lives has COVID stolen? Nature. 2022;605(7910):410–413. doi:10.1038/d41586-022-01341-7
4. Kolahchi Z, De Domenico M, Uddin LQ, et al. COVID-19 and its global economic impact. Adv Exp Med Biol. 2021;1318:825–837.
5. Ashton J. The COVID-19 pandemic: the third wave? J R Soc Med. 2021;114(7):367–368. doi:10.1177/01410768211030835
6. Cascella M, Rajnik M, Aleem A, Dulebohn SC, Di Napoli R. Features, evaluation, and treatment of coronavirus (COVID-19). In: StatPearls. Treasure Island (FL): StatPearls; 2022.
7. World Health Organization. Report of the WHO-china joint mission on coronavirus disease 2019 (COVID-19); 2020. Available from: https://www.who.int/docs/default-source/coronaviruse/who-china-joint-mission-on-covid-19-final-report.pdf.
8. Zhou F, Yu T, Du R, et al. Clinical course and risk factors for mortality of adult inpatients with COVID-19 in Wuhan, China: a retrospective cohort study. Lancet. 2020;395(10229):1054–1062. doi:10.1016/S0140-6736(20)30566-3
9. Kumar A, Arora A, Sharma P, et al. Is diabetes mellitus associated with mortality and severity of COVID-19? A meta-analysis. Diabetes Metab Syndr. 2020;14(4):535–545. doi:10.1016/j.dsx.2020.04.044
10. Simonnet A, Chetboun M, Poissy J, et al. High prevalence of obesity in severe acute respiratory syndrome coronavirus-2 (SARS-CoV-2) requiring invasive mechanical ventilation. Obesity. 2020;28(7):1195–1199. doi:10.1002/oby.22831
11. Zheng Z, Peng F, Xu B, et al. Risk factors of critical & mortal COVID-19 cases: a systematic literature review and meta-analysis. J Infect. 2020;81(2):e16–e25. doi:10.1016/j.jinf.2020.04.021
12. Cruz-Jentoft AJ, Bahat G, Bauer J, et al. Sarcopenia: revised European consensus on definition and diagnosis. Age Ageing. 2019;48(1):16–31. doi:10.1093/ageing/afy169
13. Cao L, Morley JE. Sarcopenia is recognized as an independent condition by an international classification of disease, tenth revision, clinical modification (ICD-10-CM) code. J Am Med Dir Assoc. 2016;17(8):675–677. doi:10.1016/j.jamda.2016.06.001
14. Petermann-Rocha F, Balntzi V, Gray SR, et al. Global prevalence of sarcopenia and severe sarcopenia: a systematic review and meta-analysis. J Cachexia Sarcopenia Muscle. 2022;13(1):86–99. doi:10.1002/jcsm.12783
15. Shen Y, Chen J, Chen X, Hou L, Lin X, Yang M. Prevalence and associated factors of sarcopenia in nursing home residents: a systematic review and meta-analysis. J Am Med Dir Assoc. 2019;20(1):5–13. doi:10.1016/j.jamda.2018.09.012
16. Cruz-Jentoft AJ, Sayer AA. Sarcopenia. Lancet. 2019;393(10191):2636–2646. doi:10.1016/S0140-6736(19)31138-9
17. Cruz-Jentoft AJ, Baeyens JP, Bauer JM, et al. Sarcopenia: European consensus on definition and diagnosis: report of the European Working Group on sarcopenia in older people. Age Ageing. 2010;39(4):412–423. doi:10.1093/ageing/afq034
18. Sabatino A, Cuppari L, Stenvinkel P, Lindholm B, Avesani CM. Sarcopenia in chronic kidney disease: what have we learned so far? J Nephrol. 2021;34(4):1347–1372. doi:10.1007/s40620-020-00840-y
19. Sepúlveda-Loyola W, Osadnik C, Phu S, Morita AA, Duque G, Probst VS. Diagnosis, prevalence, and clinical impact of sarcopenia in COPD: a systematic review and meta-analysis. J Cachexia Sarcopenia Muscle. 2020;11(5):1164–1176. doi:10.1002/jcsm.12600
20. Shachar SS, Williams GR, Muss HB, Nishijima TF. Prognostic value of sarcopenia in adults with solid tumours: a meta-analysis and systematic review. Eur J Cancer. 2016;57:58–67. doi:10.1016/j.ejca.2015.12.030
21. Altuna-Venegas S, Aliaga-Vega R, Maguiña JL, Parodi JF, Runzer-Colmenares FM. Risk of community-acquired pneumonia in older adults with sarcopenia of a hospital from Callao, Peru 2010–2015. Arch Gerontol Geriatr. 2019;82:100–105. doi:10.1016/j.archger.2019.01.008
22. Maeda K, Akagi J. Muscle mass loss is a potential predictor of 90-day mortality in older adults with Aspiration pneumonia. J Am Geriatr Soc. 2017;65(1):e18–e22. doi:10.1111/jgs.14543
23. Bhurchandi S, Kumar S, Agrawal S, et al. Correlation of sarcopenia with modified frailty index as a predictor of outcome in critically Ill elderly patients: a cross-sectional study. Cureus. 2021;13(10):e19065. doi:10.7759/cureus.19065
24. Weijs PJ, Looijaard WG, Dekker IM, et al. Low skeletal muscle area is a risk factor for mortality in mechanically ventilated critically ill patients. Critical Care. 2014;18(2):R12. doi:10.1186/cc13189
25. Kou HW, Yeh CH, Tsai HI, et al. Sarcopenia is an effective predictor of difficult-to-wean and mortality among critically ill surgical patients. PLoS One. 2019;14(8):e0220699. doi:10.1371/journal.pone.0220699
26. Malmstrom TK, Morley JE. SARC-F: a simple questionnaire to rapidly diagnose sarcopenia. J Am Med Dir Assoc. 2013;14(8):531–532. doi:10.1016/j.jamda.2013.05.018
27. Ida S, Kaneko R, Murata K. SARC-F for screening of sarcopenia among older adults: a meta-analysis of screening test accuracy. J Am Med Dir Assoc. 2018;19(8):685–689. doi:10.1016/j.jamda.2018.04.001
28. Kim S, Kim M, Won CW. Validation of the Korean Version of the SARC-F questionnaire to assess sarcopenia: Korean Frailty and aging cohort study. J Am Med Dir Assoc. 2018;19(1):40–45.e41. doi:10.1016/j.jamda.2017.07.006
29. Riesgo H, Castro A, Del Amo S, et al. Prevalence of risk of malnutrition and risk of sarcopenia in a reference hospital for COVID-19: relationship with mortality. Ann Nutr Metab. 2021;77(6):324–329. doi:10.1159/000519485
30. Ma Y, He M, Hou LS, et al. The role of SARC-F scale in predicting progression risk of COVID-19 in elderly patients: a prospective cohort study in Wuhan. BMC Geriatr. 2021;21(1):355. doi:10.1186/s12877-021-02310-x
31. Roberts HC, Denison HJ, Martin HJ, et al. A review of the measurement of grip strength in clinical and epidemiological studies: towards a standardised approach. Age Ageing. 2011;40(4):423–429. doi:10.1093/ageing/afr051
32. Ekiz T, Kara M, Özçakar L. Measuring grip strength in COVID-19: a simple way to predict overall frailty/impairment. Heart Lung. 2020;49(6):853–854. doi:10.1016/j.hrtlng.2020.05.011
33. Kara Ö, Kara M, Akın ME, Özçakar L. Grip strength as a predictor of disease severity in hospitalized COVID-19 patients. Heart Lung. 2021;50(6):743–747. doi:10.1016/j.hrtlng.2021.06.005
34. Tuzun S, Keles A, Okutan D, Yildiran T, Palamar D. Assessment of musculoskeletal pain, fatigue and grip strength in hospitalized patients with COVID-19. Eur J Phys Rehabil Med. 2021;57(4):653–662. doi:10.23736/S1973-9087.20.06563-6
35. Gil S, Jacob Filho W, Shinjo SK, et al. Muscle strength and muscle mass as predictors of hospital length of stay in patients with moderate to severe COVID-19: a prospective observational study. J Cachexia Sarcopenia Muscle. 2021;12(6):1871–1878. doi:10.1002/jcsm.12789
36. Gobbi M, Bezzoli E, Ismelli F, et al. Skeletal muscle mass, sarcopenia and rehabilitation outcomes in post-acute COVID-19 patients. J Clin Med. 2021;10:23. doi:10.3390/jcm10235623
37. Gonzalez MC, Barbosa-Silva TG, Heymsfield SB. Bioelectrical impedance analysis in the assessment of sarcopenia. Curr Opin Clin Nutr Metab Care. 2018;21(5):366–374. doi:10.1097/MCO.0000000000000496
38. Sipers W, Dorge J, Schols J, Verdijk LB, van Loon LJC. Multifrequency bioelectrical impedance analysis may represent a reproducible and practical tool to assess skeletal muscle mass in euvolemic acutely ill hospitalized geriatric patients. Eur Geriatr Med. 2020;11(1):155–162. doi:10.1007/s41999-019-00253-6
39. Kim D, Sun JS, Lee YH, Lee JH, Hong J, Lee JM. Comparative assessment of skeletal muscle mass using computerized tomography and bioelectrical impedance analysis in critically ill patients. Clin Nutr. 2019;38(6):2747–2755. doi:10.1016/j.clnu.2018.12.002
40. McGovern J, Dolan R, Richards C, Laird BJ, McMillan DC, Maguire D. Relation between body composition, systemic inflammatory response, and clinical outcomes in patients admitted to an urban teaching hospital with COVID-19. J Nutr. 2021;151(8):2236–2244. doi:10.1093/jn/nxab142
41. Osuna-Padilla IA, Rodríguez-Moguel NC, Rodríguez-Llamazares S, et al. Low muscle mass in COVID-19 critically-ill patients: prognostic significance and surrogate markers for assessment. Clin Nutr. 2022;41(12):2910.
42. Damanti S, Cristel G, Ramirez GA, et al. Influence of reduced muscle mass and quality on ventilator weaning and complications during intensive care unit stay in COVID-19 patients. Clin Nutr. 2021;41:2965–2972. doi:10.1016/j.clnu.2021.08.004
43. Nemec U, Heidinger B, Sokas C, Chu L, Eisenberg RL. Diagnosing sarcopenia on thoracic computed tomography: quantitative assessment of skeletal muscle mass in patients undergoing transcatheter aortic valve replacement. Acad Radiol. 2017;24(9):1154–1161. doi:10.1016/j.acra.2017.02.008
44. Derstine BA, Holcombe SA, Ross BE, Wang NC, Su GL, Wang SC. Skeletal muscle cutoff values for sarcopenia diagnosis using T10 to L5 measurements in a healthy US population. Sci Rep. 2018;8(1):11369. doi:10.1038/s41598-018-29825-5
45. Kim JW, Yoon JS, Kim EJ, et al. Prognostic implication of baseline sarcopenia for length of hospital stay and survival in patients with coronavirus disease 2019. J Gerontol a Biol Sci Med Sci. 2021;76(8):e110–e116. doi:10.1093/gerona/glab085
46. Schiaffino S, Albano D, Cozzi A, et al. CT-derived chest muscle metrics for outcome prediction in patients with COVID-19. Radiology. 2021;300(2):E328–e336. doi:10.1148/radiol.2021204141
47. Giraudo C, Librizzi G, Fichera G, et al. Reduced muscle mass as predictor of intensive care unit hospitalization in COVID-19 patients. PLoS One. 2021;16(6):e0253433. doi:10.1371/journal.pone.0253433
48. Moctezuma-Velázquez P, Miranda-Zazueta G, Ortiz-Brizuela E, et al. Low thoracic skeletal muscle area is not associated with negative outcomes in patients with COVID-19. Am J Phys Med Rehabil. 2021;100(5):413–418. doi:10.1097/PHM.0000000000001716
49. Ufuk F, Demirci M, Sagtas E, Akbudak IH, Ugurlu E, Sari T. The prognostic value of pneumonia severity score and pectoralis muscle Area on chest CT in adult COVID-19 patients. Eur J Radiol. 2020;131:109271. doi:10.1016/j.ejrad.2020.109271
50. Lundbom J. Adipose tissue and liver. J Appl Physiol. 2018;124(1):162–167. doi:10.1152/japplphysiol.00399.2017
51. Viddeleer AR, Raaphorst J, Min M, et al. Intramuscular adipose tissue at level Th12 is associated with survival in COVID-19. J Cachexia Sarcopenia Muscle. 2021;12(3):823–827. doi:10.1002/jcsm.12696
52. Yang Y, Ding L, Zou X, et al. Visceral adiposity and high intramuscular fat deposition independently predict critical illness in patients with SARS-CoV-2. Obesity. 2020;28(11):2040–2048. doi:10.1002/oby.22971
53. Feng Z, Zhao H, Kang W, et al. Association of paraspinal muscle measurements on chest computed tomography with clinical outcomes in patients with severe coronavirus disease 2019. J Gerontol a Biol Sci Med Sci. 2021;76(3):e78–e84. doi:10.1093/gerona/glaa317
54. Yi X, Liu H, Zhu L, et al. Myosteatosis predicting risk of transition to severe COVID-19 infection. Clin Nutr. 2021;41(12):3007–3015. doi:10.1016/j.clnu.2021.05.031
55. Kremer WM, Labenz C, Kuchen R, et al. Sonographic assessment of low muscle quantity identifies mortality risk during COVID-19: a prospective single-centre study. J Cachexia Sarcopenia Muscle. 2022;13(1):169–179. doi:10.1002/jcsm.12862
56. Franceschi C, Garagnani P, Parini P, Giuliani C, Santoro A. Inflammaging: a new immune-metabolic viewpoint for age-related diseases. Nat Rev Endocrinol. 2018;14(10):576–590. doi:10.1038/s41574-018-0059-4
57. Dalle S, Rossmeislova L, Koppo K. The role of inflammation in age-related sarcopenia. Front Physiol. 2017;8:1045. doi:10.3389/fphys.2017.01045
58. Bian AL, Hu HY, Rong YD, Wang J, Wang JX, Zhou XZ. A study on relationship between elderly sarcopenia and inflammatory factors IL-6 and TNF-α. Eur J Med Res. 2017;22(1):25. doi:10.1186/s40001-017-0266-9
59. Li CW, Yu K, Shyh-Chang N, et al. Circulating factors associated with sarcopenia during ageing and after intensive lifestyle intervention. J Cachexia Sarcopenia Muscle. 2019;10(3):586–600. doi:10.1002/jcsm.12417
60. Alemán H, Esparza J, Ramirez FA, Astiazaran H, Payette H. Longitudinal evidence on the association between interleukin-6 and C-reactive protein with the loss of total appendicular skeletal muscle in free-living older men and women. Age Ageing. 2011;40(4):469–475. doi:10.1093/ageing/afr040
61. Tuttle CSL, Thang LAN, Maier AB. Markers of inflammation and their association with muscle strength and mass: a systematic review and meta-analysis. Ageing Res Rev. 2020;64:101185. doi:10.1016/j.arr.2020.101185
62. Wang T. Searching for the link between inflammaging and sarcopenia. Ageing Res Rev. 2022;77:101611. doi:10.1016/j.arr.2022.101611
63. Ye Q, Wang B, Mao J. The pathogenesis and treatment of the `Cytokine Storm’ in COVID-19. J Infect. 2020;80(6):607–613. doi:10.1016/j.jinf.2020.03.037
64. Jose RJ, Manuel A. COVID-19 cytokine storm: the interplay between inflammation and coagulation. Lancet Respir Med. 2020;8(6):e46–e47. doi:10.1016/S2213-2600(20)30216-2
65. Huang C, Wang Y, Li X, et al. Clinical features of patients infected with 2019 novel coronavirus in Wuhan, China. Lancet. 2020;395(10223):497–506. doi:10.1016/S0140-6736(20)30183-5
66. Chen G, Wu D, Guo W, et al. Clinical and immunological features of severe and moderate coronavirus disease 2019. J Clin Invest. 2020;130(5):2620–2629. doi:10.1172/JCI137244
67. Xu K, Wei Y, Giunta S, Zhou M, Xia S. Do inflammaging and coagul-aging play a role as conditions contributing to the co-occurrence of the severe hyper-inflammatory state and deadly coagulopathy during COVID-19 in older people? Exp Gerontol. 2021;151:111423. doi:10.1016/j.exger.2021.111423
68. Giudice J, Taylor JM. Muscle as a paracrine and endocrine organ. Curr Opin Pharmacol. 2017;34:49–55. doi:10.1016/j.coph.2017.05.005
69. Pedersen BK, Febbraio MA. Muscles, exercise and obesity: skeletal muscle as a secretory organ. Nat Rev Endocrinol. 2012;8(8):457–465. doi:10.1038/nrendo.2012.49
70. Nelke C, Dziewas R, Minnerup J, Meuth SG, Ruck T. Skeletal muscle as potential central link between sarcopenia and immune senescence. EBioMedicine. 2019;49:381–388. doi:10.1016/j.ebiom.2019.10.034
71. Nadeau L, Aguer C. Interleukin-15 as a myokine: mechanistic insight into its effect on skeletal muscle metabolism. Appl Physiol Nutr Metab. 2019;44(3):229–238. doi:10.1139/apnm-2018-0022
72. Quinn LS, Anderson BG, Strait-Bodey L, Wolden-Hanson T. Serum and muscle interleukin-15 levels decrease in aging mice: correlation with declines in soluble interleukin-15 receptor alpha expression. Exp Gerontol. 2010;45(2):106–112. doi:10.1016/j.exger.2009.10.012
73. Pistilli EE, Quinn LS. From anabolic to oxidative: reconsidering the roles of IL-15 and IL-15Rα in skeletal muscle. Exerc Sport Sci Rev. 2013;41(2):100–106. doi:10.1097/JES.0b013e318275d230
74. O’Leary MF, Wallace GR, Bennett AJ, Tsintzas K, Jones SW. IL-15 promotes human myogenesis and mitigates the detrimental effects of TNFα on myotube development. Sci Rep. 2017;7(1):12997. doi:10.1038/s41598-017-13479-w
75. Krolopp JE, Thornton SM, Abbott MJ. IL-15 activates the Jak3/STAT3 signaling pathway to mediate glucose uptake in skeletal muscle cells. Front Physiol. 2016;7:626. doi:10.3389/fphys.2016.00626
76. Nadeau L, Patten DA, Caron A, et al. IL-15 improves skeletal muscle oxidative metabolism and glucose uptake in association with increased respiratory chain supercomplex formation and AMPK pathway activation. Biochimica Et Biophysica Acta General Subjects. 2019;1863(2):395–407. doi:10.1016/j.bbagen.2018.10.021
77. Almendro V, Busquets S, Ametller E, et al. Effects of interleukin-15 on lipid oxidation: disposal of an oral [(14)C]-triolein load. Biochim Biophys Acta. 2006;1761(1):37–42. doi:10.1016/j.bbalip.2005.12.006
78. Thornton SM, Krolopp JE, Abbott MJ. IL-15 mediates mitochondrial activity through a PPARδ-dependent-PPARα-independent mechanism in skeletal muscle cells. PPAR Res. 2016;2016:5465804. doi:10.1155/2016/5465804
79. Yalcin A, Silay K, Balik AR, Avcioğlu G, Aydin AS. The relationship between plasma interleukin-15 levels and sarcopenia in outpatient older people. Aging Clin Exp Res. 2018;30(7):783–790. doi:10.1007/s40520-017-0848-y
80. Verbist KC, Klonowski KD. Functions of IL-15 in anti-viral immunity: multiplicity and variety. Cytokine. 2012;59(3):467–478. doi:10.1016/j.cyto.2012.05.020
81. Kennedy MK, Glaccum M, Brown SN, et al. Reversible defects in natural killer and memory CD8 T cell lineages in interleukin 15-deficient mice. J Exp Med. 2000;191(5):771–780. doi:10.1084/jem.191.5.771
82. Greising SM, Mantilla CB, Gorman BA, Ermilov LG, Sieck GC. Diaphragm muscle sarcopenia in aging mice. Exp Gerontol. 2013;48(9):881–887. doi:10.1016/j.exger.2013.06.001
83. Greising SM, Medina-Martínez JS, Vasdev AK, Sieck GC, Mantilla CB. Analysis of muscle fiber clustering in the diaphragm muscle of sarcopenic mice. Muscle Nerve. 2015;52(1):76–82. doi:10.1002/mus.24641
84. Khurram OU, Fogarty MJ, Sarrafian TL, Bhatt A, Mantilla CB, Sieck GC. Impact of aging on diaphragm muscle function in male and female Fischer 344 rats. Physiol Rep. 2018;6(13):e13786. doi:10.14814/phy2.13786
85. Elliott JE, Greising SM, Mantilla CB, Sieck GC. Functional impact of sarcopenia in respiratory muscles. Respir Physiol Neurobiol. 2016;226:137–146. doi:10.1016/j.resp.2015.10.001
86. Greising SM, Mantilla CB, Medina-Martínez JS, Stowe JM, Sieck GC. Functional impact of diaphragm muscle sarcopenia in both male and female mice. Am J Physiol Lung Cell Mol Physiol. 2015;309(1):L46–52. doi:10.1152/ajplung.00064.2015
87. Cattapan SE, Laghi F, Tobin MJ. Can diaphragmatic contractility be assessed by airway twitch pressure in mechanically ventilated patients? Thorax. 2003;58(1):58–62. doi:10.1136/thorax.58.1.58
88. Laghi FA, Saad M, Shaikh H. Ultrasound and non-ultrasound imaging techniques in the assessment of diaphragmatic dysfunction. BMC Pulm Med. 2021;21(1):85. doi:10.1186/s12890-021-01441-6
89. Goligher EC, Laghi F, Detsky ME, et al. Measuring diaphragm thickness with ultrasound in mechanically ventilated patients: feasibility, reproducibility and validity. Intensive Care Med. 2015;41(4):642–649. doi:10.1007/s00134-015-3687-3
90. Dubé BP, Dres M, Mayaux J, Demiri S, Similowski T, Demoule A. Ultrasound evaluation of diaphragm function in mechanically ventilated patients: comparison to phrenic stimulation and prognostic implications. Thorax. 2017;72(9):811–818. doi:10.1136/thoraxjnl-2016-209459
91. Dres M, Demoule A. Monitoring diaphragm function in the ICU. Curr Opin Crit Care. 2020;26(1):18–25. doi:10.1097/MCC.0000000000000682
92. Tuinman PR, Jonkman AH, Dres M, et al. Respiratory muscle ultrasonography: methodology, basic and advanced principles and clinical applications in ICU and ED patients-a narrative review. Intensive Care Med. 2020;46(4):594–605. doi:10.1007/s00134-019-05892-8
93. Umbrello M, Formenti P, Longhi D, et al. Diaphragm ultrasound as indicator of respiratory effort in critically ill patients undergoing assisted mechanical ventilation: a pilot clinical study. Critical Care. 2015;19(1):161. doi:10.1186/s13054-015-0894-9
94. Corradi F, Vetrugno L, Orso D, et al. Diaphragmatic thickening fraction as a potential predictor of response to continuous positive airway pressure ventilation in Covid-19 pneumonia: a single-center pilot study. Respir Physiol Neurobiol. 2021;284:103585. doi:10.1016/j.resp.2020.103585
95. Corradi F, Isirdi A, Malacarne P, et al. Low diaphragm muscle mass predicts adverse outcome in patients hospitalized for COVID-19 pneumonia: an exploratory pilot study. Minerva Anestesiol. 2021;87(4):432–438. doi:10.23736/S0375-9393.21.15129-6
96. Malhi H. Dysphagia: warning signs and management. British Journal of Nursing. 2016;25(10):546–549. doi:10.12968/bjon.2016.25.10.546
97. Smithard D, Hansjee D, Henry D, et al. Inter-relationships between frailty, sarcopenia, undernutrition and dysphagia in older people who are admitted to acute frailty and medical wards: is there an older adult quartet? Geriatrics. 2020;5(3):41. doi:10.3390/geriatrics5030041
98. Wakabayashi H. Presbyphagia and sarcopenic dysphagia: association between aging, sarcopenia, and deglutition disorders. J Frailty Aging. 2014;3(2):97–103.
99. Wakabayashi H, Kishima M, Itoda M, et al. Diagnosis and treatment of sarcopenic dysphagia: a scoping review. Dysphagia. 2021;36(3):523–531. doi:10.1007/s00455-021-10266-8
100. Campo-Rivera N, Ocampo-Chaparro JM, Carvajal-Ortiz R, Reyes-Ortiz CA. Sarcopenic dysphagia is associated with mortality in institutionalized older adults. J Am Med Dir Assoc. 2022;23(10):1720.e1711–1720.e1717. doi:10.1016/j.jamda.2022.06.016
101. Miles A, McRae J, Clunie G, et al. An international commentary on dysphagia and dysphonia during the COVID-19 pandemic. Dysphagia. 2022;37(6):1349–1374. doi:10.1007/s00455-021-10396-z
102. Regan J, Walshe M, Lavan S, et al. Post-extubation dysphagia and dysphonia amongst adults with COVID-19 in the Republic of Ireland: a prospective multi-site observational cohort study. Clin Otolaryngol. 2021;46(6):1290–1299. doi:10.1111/coa.13832
103. Zuercher P, Lang B, Moser M, Messmer AS, Waskowski J, Schefold JC. Dysphagia incidence in intensive care unit patients with coronavirus disease 2019: retrospective analysis following systematic dysphagia screening. J Laryngol Otol. 2022;136(12):1278–1283. doi:10.1017/S0022215122001517
104. Naseeb MA, Volpe SL. Protein and exercise in the prevention of sarcopenia and aging. Nutr Res. 2017;40:1–20. doi:10.1016/j.nutres.2017.01.001
105. Tieland M, Dirks ML, van der Zwaluw N, et al. Protein supplementation increases muscle mass gain during prolonged resistance-type exercise training in frail elderly people: a randomized, double-blind, placebo-controlled trial. J Am Med Dir Assoc. 2012;13(8):713–719. doi:10.1016/j.jamda.2012.05.020
106. Tieland M, van de Rest O, Dirks ML, et al. Protein supplementation improves physical performance in frail elderly people: a randomized, double-blind, placebo-controlled trial. J Am Med Dir Assoc. 2012;13(8):720–726. doi:10.1016/j.jamda.2012.07.005
107. Dent E, Morley JE, Cruz-Jentoft AJ, et al. International clinical practice guidelines for sarcopenia (ICFSR): screening, diagnosis and management. J Nutr Health Aging. 2018;22(10):1148–1161. doi:10.1007/s12603-018-1139-9
108. Bauer J, Morley JE, Schols A, et al. Sarcopenia: a time for action. An SCWD position paper. J Cachexia Sarcopenia Muscle. 2019;10(5):956–961. doi:10.1002/jcsm.12483
109. Moonen HP, Hermans AJ, Jans I, van Zanten AR. Protein requirements and provision in hospitalised COVID-19 ward and ICU patients: agreement between calculations based on body weight and height, and measured bioimpedance lean body mass. Clin Nutr ESPEN. 2022;49:474–482. doi:10.1016/j.clnesp.2022.03.001
110. Barazzoni R, Bischoff SC, Breda J, et al. ESPEN expert statements and practical guidance for nutritional management of individuals with SARS-CoV-2 infection. Clin Nutr. 2020;39(6):1631–1638. doi:10.1016/j.clnu.2020.03.022
111. Martindale R, Patel JJ, Taylor B, Arabi YM, Warren M, McClave SA. Nutrition therapy in critically ill patients with coronavirus disease 2019. JPEN J Parenter Enteral Nutr. 2020;44(7):1174–1184. doi:10.1002/jpen.1930
112. Latham CM, Brightwell CR, Keeble AR, et al. Vitamin D promotes skeletal muscle regeneration and mitochondrial health. Front Physiol. 2021;12:660498. doi:10.3389/fphys.2021.660498
113. Beaudart C, Buckinx F, Rabenda V, et al. The effects of vitamin D on skeletal muscle strength, muscle mass, and muscle power: a systematic review and meta-analysis of randomized controlled trials. J Clin Endocrinol Metab. 2014;99(11):4336–4345. doi:10.1210/jc.2014-1742
114. Murai IH, Fernandes AL, Sales LP, et al. Effect of a single high dose of vitamin D3 on hospital length of stay in patients with moderate to severe COVID-19: a randomized clinical trial. JAMA. 2021;325(11):1053–1060. doi:10.1001/jama.2020.26848
115. Dissanayake HA, de Silva NL, Sumanatilleke M, et al. Prognostic and therapeutic role of vitamin D in COVID-19: systematic review and meta-analysis. J Clin Endocrinol Metab. 2022;107(5):1484–1502. doi:10.1210/clinem/dgab892
116. Lakkireddy M, Gadiga SG, Malathi RD, et al. Impact of daily high dose oral vitamin D therapy on the inflammatory markers in patients with COVID 19 disease. Sci Rep. 2021;11(1):10641. doi:10.1038/s41598-021-90189-4
117. Entrenas Castillo M, Entrenas Costa LM, Vaquero Barrios JM, et al. Effect of calcifediol treatment and best available therapy versus best available therapy on intensive care unit admission and mortality among patients hospitalized for COVID-19: a pilot randomized clinical study. J Steroid Biochem Mol Biol. 2020;203:105751. doi:10.1016/j.jsbmb.2020.105751
118. NICE. National institute for health and care excellence: guidelines. In: COVID-19 Rapid Guideline: Vitamin D. London: National Institute for Health and Care Excellence (NICE); 2020.
119. Cruz-Jentoft AJ, Landi F, Schneider SM, et al. Prevalence of and interventions for sarcopenia in ageing adults: a systematic review. Report of the International Sarcopenia Initiative (EWGSOP and IWGS). Age Ageing. 2014;43(6):748–759. doi:10.1093/ageing/afu115
120. Leenders M, Verdijk LB, van der Hoeven L, van Kranenburg J, Nilwik R, van Loon LJ. Elderly men and women benefit equally from prolonged resistance-type exercise training. J Gerontol a Biol Sci Med Sci. 2013;68(7):769–779. doi:10.1093/gerona/gls241
121. Felten-Barentsz KM, van Oorsouw R, Klooster E, et al. Recommendations for hospital-based physical therapists managing patients with COVID-19. Phys Ther. 2020;100(9):1444–1457. doi:10.1093/ptj/pzaa114
122. Wang PY, Li Y, Wang Q. Sarcopenia: an underlying treatment target during the COVID-19 pandemic. Nutrition. 2021;84:111104. doi:10.1016/j.nut.2020.111104
123. da Silveira MP, da Silva Fagundes KK, Bizuti MR, Starck É, Rossi RC, de Resende ESDT. Physical exercise as a tool to help the immune system against COVID-19: an integrative review of the current literature. Clin Exp Med. 2021;21(1):15–28. doi:10.1007/s10238-020-00650-3
124. Jones SA, Hunter CA. Is IL-6 a key cytokine target for therapy in COVID-19? Nat Rev Immunol. 2021;21(6):337–339. doi:10.1038/s41577-021-00553-8
125. Hunter CA, Jones SA. IL-6 as a keystone cytokine in health and disease. Nat Immunol. 2015;16(5):448–457. doi:10.1038/ni.3153
126. Smolen JS, Landewé RBM, Bijlsma JWJ, et al. EULAR recommendations for the management of rheumatoid arthritis with synthetic and biological disease-modifying antirheumatic drugs: 2019 update. Ann Rheum Dis. 2020;79(6):685–699. doi:10.1136/annrheumdis-2019-216655
127. Shankar-Hari M, Vale CL, Godolphin PJ, et al. Association between administration of IL-6 antagonists and mortality among patients hospitalized for COVID-19: a meta-analysis. JAMA. 2021;326(6):499–518. doi:10.1001/jama.2021.11330
128. World Health Organization. Update to living WHO guideline on drugs for covid-19. BMJ. 2022;378:o2224. doi:10.1136/bmj.o2224
129. Muñoz-Cánoves P, Scheele C, Pedersen BK, Serrano AL. Interleukin-6 myokine signaling in skeletal muscle: a double-edged sword? FEBS J. 2013;280(17):4131–4148. doi:10.1111/febs.12338
130. Tsujinaka T, Fujita J, Ebisui C, et al. Interleukin 6 receptor antibody inhibits muscle atrophy and modulates proteolytic systems in interleukin 6 transgenic mice. J Clin Invest. 1996;97(1):244–249. doi:10.1172/JCI118398
131. Fujita R, Kawano F, Ohira T, et al. Anti-interleukin-6 receptor antibody (MR16-1) promotes muscle regeneration via modulation of gene expressions in infiltrated macrophages. Biochim Biophys Acta. 2014;1840(10):3170–3180. doi:10.1016/j.bbagen.2014.01.014
132. Kostek MC, Nagaraju K, Pistilli E, et al. IL-6 signaling blockade increases inflammation but does not affect muscle function in the mdx mouse. BMC Musculoskelet Disord. 2012;13:106. doi:10.1186/1471-2474-13-106
133. Tournadre A, Pereira B, Dutheil F, et al. Changes in body composition and metabolic profile during interleukin 6 inhibition in rheumatoid arthritis. J Cachexia Sarcopenia Muscle. 2017;8(4):639–646. doi:10.1002/jcsm.12189
134. Suzuki R, Yamasoba D, Kimura I, et al. Attenuated fusogenicity and pathogenicity of SARS-CoV-2 Omicron variant. Nature. 2022;603(7902):700–705. doi:10.1038/s41586-022-04462-1
135. Aleem A, Akbar Samad AB, Slenker AK. Emerging variants of SARS-CoV-2 and novel therapeutics against coronavirus (COVID-19). In: StatPearls. Treasure Island (FL): StatPearls Publishing. StatPearls Publishing LLC; 2022.
© 2023 The Author(s). This work is published and licensed by Dove Medical Press Limited. The
full terms of this license are available at https://www.dovepress.com/terms.php
and incorporate the Creative Commons Attribution
- Non Commercial (unported, v3.0) License.
By accessing the work you hereby accept the Terms. Non-commercial uses of the work are permitted
without any further permission from Dove Medical Press Limited, provided the work is properly
attributed. For permission for commercial use of this work, please see paragraphs 4.2 and 5 of our Terms.
Recommended articles

Resveratrol Prevents Skeletal Muscle Atrophy and Senescence via Regulation of Histone Deacetylase 2 in Cigarette Smoke-Induced Mice with Emphysema
Li C, Deng Z, Zheng G, Xie T, Wei X, Huo Z, Bai J
Journal of Inflammation Research 2022, 15:5425-5437
Published Date: 16 September 2022

Anorexia of Aging: Metabolic Changes and Biomarker Discovery
Picca A, Calvani R, Coelho-Júnior HJ, Landi F, Marzetti E
Clinical Interventions in Aging 2022, 17:1761-1767
Published Date: 2 December 2022
Hematological, Inflammatory, Coagulation, and Oxidative/Antioxidant Biomarkers as Predictors for Severity and Mortality in COVID-19: A Prospective Cohort-Study
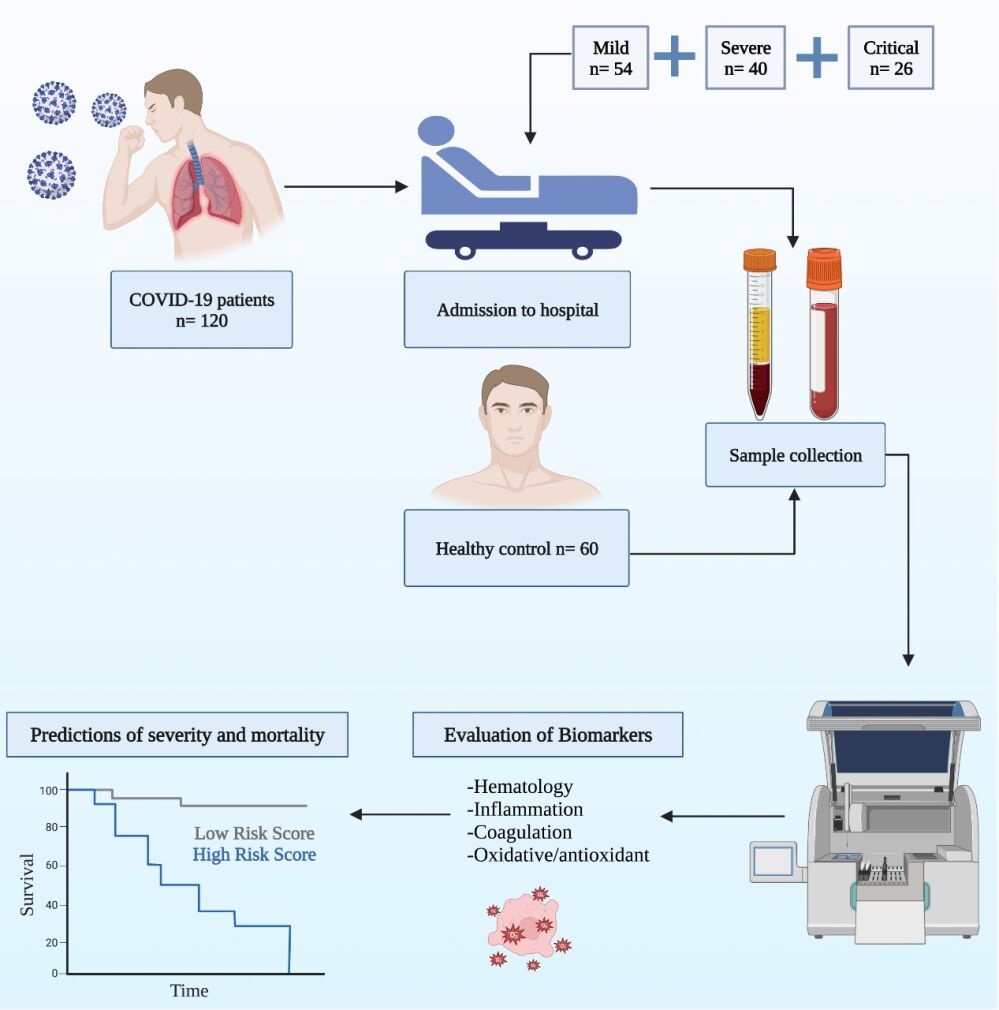
Smail SW, Babaei E, Amin K
International Journal of General Medicine 2023, 16:565-580
Published Date: 17 February 2023
The Diagnostic Value of Inflammatory Markers (CRP, IL6, CRP/IL6, CRP/L, LCR) for Assessing the Severity of COVID-19 Symptoms Based on the MEWS and Predicting the Risk of Mortality
Wolszczak-Biedrzycka B, Dorf J, Milewska A, Łukaszyk M, Naumnik W, Kosidło JW, Dymicka-Piekarska V
Journal of Inflammation Research 2023, 16:2173-2188
Published Date: 22 May 2023

The Association Between Sarcopenia and Diabetes: From Pathophysiology Mechanism to Therapeutic Strategy
Chen H, Huang X, Dong M, Wen S, Zhou L, Yuan X
Diabetes, Metabolic Syndrome and Obesity 2023, 16:1541-1554
Published Date: 30 May 2023