Back to Journals » Journal of Inflammation Research » Volume 16
Neural Stem Cell-Derived Exosomes Improve Neurological Function in Rats with Cerebral Ischemia-Reperfusion Injury by Regulating Microglia-Mediated Inflammatory Response
Authors Zhao X, Zhu J , Chen S , Liu R, Long T
Received 1 May 2023
Accepted for publication 8 July 2023
Published 24 July 2023 Volume 2023:16 Pages 3079—3092
DOI https://doi.org/10.2147/JIR.S414121
Checked for plagiarism Yes
Review by Single anonymous peer review
Peer reviewer comments 2
Editor who approved publication: Dr Monika Sharma
Xue Zhao,* Junde Zhu, Shan Chen,* Ruojing Liu, Tingting Long
Department of Human Anatomy, School of Basic Medicine, Guizhou Medical University, Guiyang, People’s Republic of China
*These authors contributed equally to this work
Correspondence: Junde Zhu, Department of Human Anatomy, School of Basic Medicine, Guizhou Medical University, Guian New Area, Guiyang, 550025, People’s Republic of China, Tel +86-851-88416251, Email [email protected]
Purpose: To investigate the effect of neural stem cell-derived exosomes (NSC-Exos) on neural function after rat cerebral ischemia-reperfusion injury by regulating microglia-mediated inflammatory response.
Methods: SD rats were randomly divided into Sham group, IRI group, PBS group and NSC-Exos group. Each group was divided into 1d, 3d, 7d and 14d subgroups. In the Sham group, only cervical vessels were isolated without blockage. MCAO model was constructed in the other three groups by blocking middle cerebral artery with thread embolism. PBS group and NSC-Exos group were, respectively, injected into the lateral ventricle of PBS and Exos. Neurobehavioral deficit scores were performed for each subgroup at relative time points, then brains were taken for TTC staining, parietal cortex histopathology and microglia-mediated inflammatory response-related factors were detected.
Results: Compared with Sham group, neurological defect score and infarction volume in both the IRI and PBS groups were significantly increased. The exploration target quadrant time and escape latency time of maze test were increased. The number of CD86+/Iba1+ double-positive cells increased, while CD206+/Iba1+ double-positive cells decreased. The expressions of IL-6 and CD86 in parietal cortex were increased, while the expressions of Arg1 and CD206 were decreased. Compared with the IRI group and PBS group, neurological defect score and infarction volume in NSC-Exos group were decreased. The exploration target quadrant time and escape latency time of water maze test were decreased. The number of CD206+/Iba1+ double-positive cells increased, while CD86+/Iba1+ double-positive cells decreased. The expressions of Arg1 and CD206 in parietal cortex were increased, while the expressions of IL-6 and CD86 were decreased.
Conclusion: NSC-Exos can promote the polarization of microglia, that is, inhibit the polarization of M1 and promote polarization of M2, reduce microglia-mediated neuroinflammation, suggesting that NSC-Exos may be a strategy for the treatment of microglia-mediated neuroinflammation after ischemic brain injury.
Keywords: cerebral ischemia, neural stem cell, exosome, microglia, neuroinflammation
Graphical Abstract:
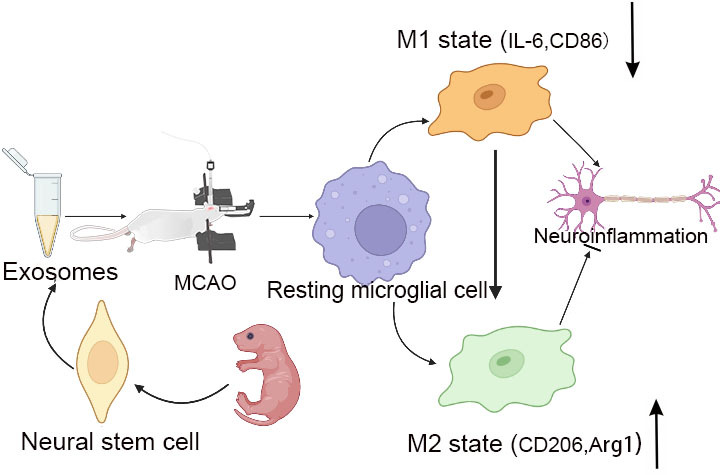
Introduction
Ischemic stroke is a common acute cerebrovascular disease, it always been a major cause of death and disability worldwide.1,2 Due to the complex pathophysiological mechanisms of stroke, which has great difficulties in treatment and prevention. Microglia are the resident immune cells of central nervous system, mainly initiate inflammation and immune response.3 Microglia are activated, rapidly migrate to the injury site and play a crucial role in neuroinflammation following stroke.4 Indeed, mounting evidence demonstrated that the role of microglia in stroke was based on their two polarizations: the pro-inflammatory (M1 phenotype) and anti-inflammatory (M2 phenotype).5,6 Regulating the polarization of microglia, especially promoting the anti-inflammatory (M2 phenotype) transformation during the recovery period of stroke, may be a novel therapeutic strategy for post-stroke neurological recovery.
Neural stem cells (NSCs) are a kind of cell population with multi-directional differentiation potential and self-renewal abilities.7 Due to low immunogenicity and wide sources, stem cells have received more and more attention and provide new directions for future clinical treatment of diseases.8 In recent years, some works have shown that the therapeutic effect of neural stem cells is more dependent on the paracrine method, while exosomes (exos) are critical paracrine mediators.9 Recently, exosomes have been identified as a crucial role in intercellular communication by delivering proteins, RNAs and miRNAs to neighboring cells.10,11 Although some studies show that neural stem cell-derived exosomes (NSC-Exos) are powerful mediators obtained in neuronal protective and immune response under multiple pathological conditions,12 little is known about their role in microglial activation after stroke. In the present study, the middle cerebral artery occlusion (MCAO) model was used to examine the efficacy of NSC-Exos on modulating microglial polarization and focused on whether NSC-Exos facilitated neurological recovery in an anti-inflammatory phenotype polarization-dependent manner.
Materials and Methods
Animals
12–14 weeks male Sprague-Dawley (SD) rats, body weight 250–280g. Reared seven days in advance to adapt to the environment (temperature 22–24°C; humidity 40–70%; 12-hour light/dark cycle). SD rats were purchased from the Experimental Animal Center of Guizhou Medical University, China. [License No. SYXK (Qian) 2018–0001]. All experimental procedures were approved by the Ethics Committee of Guizhou Medical University (Approval No. 2100034), according to the Laboratory Animal-Guideline for Ethical Review of Animal Welfare (Reference NO. GB/T 35892–2018).
Experimental Groups and Animal Models
SD rats were randomly divided into Sham group, IRI group (Ischemia-reperfusion injury, IRI), PBS group (Phosphate Buffered Saline, treatment) and NSC-Exos group (IRI+NSC-Exos treatment). Each group was further divided into four subgroups: 1d, 3d, 7d, and 14d (n = 10). Except for the Sham group, the other groups were established as previously described by Zea-Longa’s method.13 Briefly, rats were anesthetized intraperitoneally with pentobarbital (30 mg/kg). Made a slight rightward incision along the neck to expose right common carotid artery (CCA), internal carotid artery (ICA) and external carotid artery (ECA). A small incision was made about 3–5mm below the ECA end, entered nylon suture along the incision to block ICA until slight resistance was felt, the insertion depth was about 20 ± 0.5mm. After 60 minutes (min), the nylon suture was pulled out to restore blood flow perfusion, and the postoperative situation was observed until awakening.
Lateral Ventricle Administration
Rats were fixed on a stereotaxic instrument, and the skin incision was about 1.5–2.0cm in the midsagittal line. Adopted the bregma as the coordinate zero point, 0.5mm behind the bregma, 2.0mm to the right of the midline, and 4.5mm in depth, simultaneously determined the correctness by withdrawing the cerebrospinal fluid with the micro syringe.14 The NSC-Exos group was injected with NSC-Exos (10μg), while the PBS group was injected with PBS (10μL). All injections were performed slowly at a rate of 2.0 μL/min, and the syringe was withdrawn slowly after 10 min. Lateral ventricle injection followed the aseptic principle and recommended procedures to avoid complications.
Co-Localization of Fluorescent Probe PKH26 Labeling and MAP-2 Neurons
NSC-Exos was labeled with the red fluorescent dye PKH26 (Umibio Co. Ltd, China), and the dye working solution was prepared with the PKH26 linker of component A and the Diluent C of component B in the dark. Add 50μL working solution to about 200μg exosomes, then add about 10mL PBS to the exosome-dye complex. Centrifuge again to remove excess dye, and the resulting pellet was labeled exosomes. Immunofluorescent staining was performed on the brain slices (NSC-Exos group) to detect the co-localization of PKH26 labeling and MAP-2 nerve cells, the antibody used was MAP-2 (Boster, China).
Neurobehavioral Assessment
In this study, Longa’s scoring method13 was conducted to evaluate neurological function on 1d, 3d, 7d, and 14d after MCAO, and scores were evaluated by an observer blinded to each group. Score 0: normal activity of rats without obvious nerve injury. Score 1: left forelimb failed to extend (mild neurological deficit). Score 2: rats walked turning in circles to the left (moderate neurological deficit). Score 3: rats spontaneously turned to the left (severe neurological deficit). Score 4: rats lost consciousness and could not walk. Rats with a scoring standard of ≥1 were included in this study, unqualified rats were excluded, and subsequent experiments were made in the same batch to supplement the number of each group.
Balance Test
Rats were placed on a wooden bar, and a sponge was placed under the wooden bar to prevent the rats from falling. Start timing when rats were stood firmly at the marked position, then used a camera to record the situation on the wooden bar for 120s. Score 0: passed the wooden bar smoothly. Score 1: grasped the edge without falling. Score 2: grasped the wooden bar, but one foot fell off. Score 3: grasped the wooden bar, both feet fell or turned over from the bars (>120s). Score 4: tried to keep steady but finally fell (>90s). Score 5: tried to keep steady but finally fell (>60s). Score 6: unable to keep stable, directly fell (<30s). Each rat was repeated three times to get the average value.
Morris Water Maze
Morris water maze (MWM) test was conducted to assess spatial learning and memory capability of MCAO rats as previously described.15 Briefly, rats were put into a black water tank and filled with water (diameter 150cm; height 60cm; temperature 22 ± 1.0°C). Morris software circled the edges and measured the corresponding quadrants with the computer. An escape platform (diameter 10cm) was placed in the middle of the quadrant, and this position remained constant throughout the experiment. Four experiments were carried out from the first day to the fourth day, rats were placed in the tank, and facing the wall to explore freely every day. If the platform was found within 60s, the time was the escape latency period (end). If not found, guide them to stand on the platform and stay for 10s to end the experiment. The fifth day was the space exploration experiment, removing the platform from the tank and let it explore freely. Finally, the escape latency, target quadrant exploration time, and movement trajectory were recorded within the specified time to represent the memory function. Data were analyzed using the Morris water maze image process system (Chengdu Tai Meng Technology, China).
Measurement of Infarct Volume
Rats were stained with 2,3,5-triphenyl tetrazolium chloride (TTC, Boster, China) to evaluate the degree of cerebral infarction. At 3d after reperfusion, the rats were euthanized with a lethal dose of pentobarbital (100mg/kg). The brain tissues were removed immediately, put in −20°C for about 30 min, and sliced coronally into 2mm-thick sections. Sections were incubated in 2% TTC solution for nearly 20 min at room temperature and then immersed into 4% paraformaldehyde (PFA) solution overnight for fixation. As a result, normal areas were stained red, while infarcted areas appeared a pale gray color. ImageJ calculated the infarct area according to the following formula: infarct size (%) = (contralateral hemisphere area − ipsilateral non-infarct area)/contralateral area × 100%.
Immunohistochemistry Staining
Iba1, a characteristic marker of microglia, was stained in the parietal cortex of the brain. Immunohistochemical staining was carried out using the avidin-biotin method and SABC kit (Boster, China). Deparaffinized sections were treated with 3% hydrogen peroxide for 15 min before conducting antigen retrieval using a microwave oven at 98°C for 10 min. After washing steps, incubated with bovine serum albumin (BSA) for 30 min to block nonspecific antigen binding. Subsequently, sections were incubated with anti-Iba1 (Bioss, China) at 4°C for 12h. After washing steps, a biotin-marked secondary antibody was applied for 20 min followed by a peroxidase-marked streptavidin for an additional 20 min. The reactions were visualized by using 3,3’-diaminobenzidine tetrahydrochloride (DAB, Solarbio, China). Cell nuclei were counterstained with hematoxylin. Finally, taken pictures under the microscope and calculated the number of Iba1+ cells.
Immunofluorescence Staining
After preparing paraffin sections, the slides were incubated with a series of primary antibodies, including Iba1, CD86 (Zen-bio, China), and CD206 (Proteintech, China). Cell nuclei were stained with 4′,6-diamidino-2-phenylindole (DAPI, Cell Signaling, USA). Finally, the sections were imaged with a laser confocal microscope (IXplore SPinSR10, Olympus, Japan) and quantified with the ImageJ software. ImageJ circled the region of interest (ROI), counted the co-labeled cells within ROI area, then determined the percentage of co-labeled cells. The result was expressed as a percentage: co-labeled cells (100%) = (total number of double positive cells/total number of Iba1+ cells × 100%).16
Western Blot Analysis
Rats were euthanized with a lethal dose of pentobarbital, and the right parietal cortex was separated and weighed. Put the brain tissue into the pre-cooled protein lysate for total protein extraction, all steps were performed on ice. After loading an equal amount of protein onto the gel, the electrophoresis procedure was initiated. The proteins were then transferred onto polyvinylidene fluoride (PVDF, Solarbio, China) membranes and blocked with 5% bovine serum albumin (BSA) for 2h. Next, membranes were incubated with primary antibodies at 4°C for 12h. After washing three times, the membranes were incubated with secondary antibody (Boster, China) at room temperature for 2h. The primary antibodies used in this study were as follows: CD86, CD206, IL-6 (Bioss, China), and Arg1 (Ptm-bio, China), with β-actin (Bioss, China) as the internal reference. Finally, the Western blot detection system (Bio-rad, USA) was used to observe protein signals, which were quantified by ImageJ.
Real-Time PCR Analysis
Rats were euthanized with a lethal dose of pentobarbital, the brain tissue was weighed and grinded, and then extracted the total RNA with Trizol (Beyotime, China). cDNA was synthesized from RNA by reverse transcription using a M5 Sprint qPCR RT kit (Mei5bio, China). The reaction was performed on a real-time PCR system (Bio-rad, USA). The reaction procedure: 95°C, ➡30s, 95°C, ➡10s, 60°C, ➡30s, 40 cycles. The comparative mRNA expressed level was normalized to the GAPDH and calculated according to the 2−ΔΔCt method. Primers were synthesized by Sangon Biotech (Shanghai, China), and the primer sequences were as follows: CD86 (F:5’-GATTGCAGGTCCCAGTTCACTTC-3’, R:5’-CCACTGTCCTGCTTGGACTCAC-3’); CD206 (F:5’-TGGAGTGGCAGGTG GTTTATG-3’, R:5’-GGTTCAGGAGTTGTTGTGGGC-3’); Arg1 (F:5’-ATCGGAGC GCCTTTCTCAAA-3’, R:5’-GCAGATTCCCAGAGCTGGTT-3’); GAPDH (F:5’-AG ACAGCCGCATCTTCTTGT-3’, R:5’-TACTCAGCACCAGCATCACC-3’).
Statistical Analysis
All data were expressed as mean ± standard deviation and analyzed using SPSS 20.0 software (SPSS Inc., Chicago, USA). A one-way analysis of variance followed by Student-Newman-Keuls test was used to assess statistical significance. P<0.05, the statistical significance of the difference was considered feasible.
Results
NSC-Exos Alleviate Neurobehavioral Deficits After MCAO
Neurobehavioral deficits were evaluated using Longa’s score and balance beam test. The scores further showed that NSC-Exos treatment greatly attenuated neurobehavioral deficits after stroke compared to the IRI and PBS group (P<0.05). There was no significant difference between the IRI and PBS group (P>0.05). However, the NSC-Exos group exhibited significantly lower scores on 1d, 3d, 7d, and 14d after MCAO compared with the IRI and PBS groups (P<0.05, Figure 1A and B).
NSC-Exos Improve the Cognitive Deficits in Rats After MCAO
To further investigate the neuroprotective effects of NSC-Exos, spatial learning and cognitive function were assessed by MWM test.17 In the MWM analysis, the IRI and PBS groups showed substantial spatial learning ability loss and memory dysfunction as indicated by the longer escape latency time within 5 training days (Figure 2A) and longer target platform quadrant time (Figure 2B), and movement trajectories were disordered (Figure 2C) compared with the sham group (P<0.05). In contrast, the NSC-Exos group showed a significant spatial learning ability and memory function, NSC-Exos treatment reduced the escape latency time (Figure 2A) and target quadrant time (Figure 2B), and movement trajectory was clear compared with the IRI and PBS groups (P<0.05, Figure 2C). Based on our results, we concluded that NSC-Exos could improve the neurological outcome after MCAO.
NSC-Exos Reduce the Infarct Size in Rats After MCAO
TTC staining was used to observe the infarction, the normal tissue was red, while the infarct area was white. The results showed that brain tissue of the sham group was red and there was no obvious white infarct area, while the IRI, PBS and NSC-Exos groups had infarct areas, mainly concentrated in the hippocampus, frontal cortex, and parietal cortex (Figure 3A). Besides, NSC-Exos group significantly reduced infarct area compared with the IRI and PBS groups (P<0.05, Figure 3B). Based on the results, we concluded that NSC-Exos could reduce infarct area and alleviate neurobehavioral deficits after MCAO. We next examined the effect of NSC-Exos in ischemic rats, PKH26-labeled exosomes were detected in the lateral ventricle of ischemic rats. Images showed that PKH26-labeled exosomes (red) were localized in the cytoplasm of MAP-2+ neurons (pink), and a small amount overlapped with the nucleus, indicating exosomes were successfully taken up by neurons (Figure 4).18
NSC-Exos Attenuate Microglia and Inflammation After MCAO
To understand NSC-Exos effects on stroke recovery, we examined the expression of microglia at 1d, 3d, 7d, and 14d after stroke through immunohistochemical staining. For observing microglia, we applied Iba1 antibody. The morphology of Iba1 showed a highly branched appearance and hypertrophic somatic cells in the IRI, PBS group (Figure 5A). NSC-Exos treatment reduced the number of branches and somatic cells compared with the IRI and PBS groups (P<0.05, Figure 5A), in addition to inhibit the density of microglia. In the quantified data, we found that microglia were markedly increased in inflammatory response, and NSC-Exos treatment was able to reverse the elevated microglial level. In addition, the number of Iba1+ cells was significantly reduced following NSC-Exos treatment (Figure 5B).
NSC-Exos Shift Microglial Polarization Toward M2 Phenotype
Microglia were activated from a resting state to an active state after MCAO.19 Iba1 is a type of calcium-binding protein specifically expressed by microglia in the central nervous system, and CD86 and CD206 are one of the markers of M1 and M2.20 Therefore, M1/M2 markers and Iba1 in the parietal cortex of rats were observed on 1d, 3d, 7d, and 14d after cerebral ischemia. CD86+/Iba1+ and CD206+/Iba1+ immunofluorescence co-staining were used to detect M1 and M2 phenotypes, respectively (Figures 6 and 7). Compared with the sham group, the number of CD86+/Iba1+ cells (M1 microglia) and CD206+/Iba1+ cells (M2 microglia) in ischemia parietal cortex significantly increased in the IRI and PBS groups at 1d, 3d, 7d, and 14d (P<0.05, Figures 6 and 7), and most of them expressed the CD86+/Iba1+ especially at 1d and 3d (Figure 6A, B, E and F). However, the number of CD86+/Iba1+ cells was statistically reduced in rats treated with NSC-Exos (Figure 6A–D and E–H), while the number of CD206+/Iba1+ cells was significantly increased when compared with the IRI and PBS groups (Figure 7A–D and E–H), and the CD206+/Iba1+ cells continued to increase at 1d, 3d, and 7d (Figure 7A–C and E–G), reaching the maximum at 14d (Figure 7D and H).
NSC-Exos Ameliorate Inflammatory Microenvironment After MCAO
The effect of NSC-Exos was further validated by determining inflammatory factors. The levels of M1-associated factors (IL-6, CD86) and M2-associated factors (CD206, Arg1) in parietal cortex were detected by Western blot analysis at 1d, 3d, 7d, and 14d (Figure 8). Notably, compared with sham group, the levels of IL-6, CD86 were obviously increased at 1d, 3d, 7d, and 14d after stroke. NSC-Exos treatment significantly reduced the IL-6 and CD86 (Figure 8A, B and E–F) and increased CD206 and Arg1 (Figure 8C, D, G and H) at 1d, 3d, 7d, 14d in MACO rats compared with IRI and PBS groups. Indicating an amelioration of local inflammatory microenvironment mediated by NSC-Exos in parietal cortex. Similar to the Western blot analysis, mRNA levels in the ischemic tissue were determined at 3d after stroke (Figure 8I–K). Results showed that the NSC-Exos treatment significantly reduced the level of CD86 (Figure 8I) and increased the level of CD206 and Arg1 (Figure 8J and K). Accordingly, these data favor the potential of NSC-Exos treatment in improving inflammation and attenuating tissue damage.
Discussion
In the present study, we identified that NSC-Exos had neuroprotective effects on cerebral ischemia/reperfusion injury, which were mainly reflected in the following aspects: (1) NSC-Exos reduced infarction size, brain edema and neuroinflammation, and ameliorated neurological deficit in MCAO/R SD rats; (2) PKH26-labeled exosomes have entered the site of cerebral damage and exerted neuroprotective effects; (3) NSC-Exos regulated the secretion of microglia-related inflammatory factors, thereby alleviated the occurrence of inflammation. Furthermore, we found that neuroprotective effect of NSC-Exos was mediated through promoting the transition of microglia to an anti-inflammatory phenotype.
Microglia, as the main resident immune cells, display a beneficial efficacy in ameliorating inflammatory microenvironment for stroke via shifting polarization from pro-inflammatory phenotype to anti-inflammatory phenotype.21 With the fast pace of stem cell research, increasing numbers of stem cells have been associated with specific microglia polarization in improving stroke disease.22 However, due to low survival rate after stem cell transplantation, the clinical application was still limited. Exos are an emerging therapeutic modality with advantages, including reduced tumorigenicity, low immunogenicity, and enhanced cross of blood–brain barrier, and it has been shown to play a role in a variety of central system diseases.23 Thus, NSC-Exos based therapies are emerging as ideal candidates for functional recovery in stroke treatment. This study explored how NSC-Exos regulate microglia polarization to inhibit the occurrence of inflammatory response, providing a new therapeutic method for stroke.
To better observe whether NSC-Exos can reach the site of injury and exert their effects, we labeled them with a PKH26 probe. PKH26-labeled Exos not only allow us to discover distribution in vivo but also differentiate them from other cells or extracellular particles. Studies have reported that Exos can be labeled with lipophilic fluorescent dye PKH26 and stably inserted into lipid domains of recipient cell membranes, with no significant effect on the survival and other physiological characteristics of target cells.24 Finally, infiltration of PKH26-labeled intraventricular Exos into the site of brain injury was demonstrated by fluorescence microscopy. Co-localization expression of the PKH26 probe with MAP-2 positive cells in rat parietal cortex was observed, indicating that Exos can be successfully taken up by nerve cells, which is beneficial for monitoring in subsequent experiments. In some animal experiments, it has been shown that therapeutic effect of NSC-Exos can be supported by improving symptoms of stroke, reducing infarct volume, and increasing neuroprotective effects.25 Our current study further supported the anti-inflammatory effect of NSC-Exos in ischemic stroke, for example, the effect of NSC-Exos treatment improved neurological deficits and reduced relative infarct volume in stroke rats. It was indicated that NSC-Exos have a good intervention effect, and these results are consistent with a study by Zhu et al26 Considering the dual effects of inflammatory response after stroke which may be microglial polarization, the neuroprotective effect of NSC-Exos through regulating microglia-induced inflammation warranted investigation in the future.
To further elucidate polarization of microglia, immunofluorescence staining was further used to confirm the effect of NSC-Exos on modulating microglial polarization. In the present study, we found that the number of CD86+/Iba1+ and CD206+/Iba1+ cells in parietal cortex increased after stroke. However, after intervention with NSC-Exos, the number of CD86+/Iba1+ cells decreased and CD206+/Iba1+ cells increased, suggesting that NSC-Exos treatment can promote microglia to M2 phenotype in stroke, which is consistent with Luo et al studies.27 Furthermore, we characterized that NSC-Exos treatment could alleviate neuroinflammation after stroke, reduce the levels of inflammatory cytokines such as IL-6 and CD86, and increase the levels of Arg1 and CD206. Together, these data further supported that NSC-Exos promoted post-stroke microglia to the anti-inflammatory phenotype. However, the spatiotemporal expression and peak time of inflammatory factors are different from other reports. Hu et al found that pro-inflammatory factors were no significant change in 1d, but their expression gradually increased from 3d onwards, whereas anti-inflammatory factors were induced to express from 1d to 3d and peaked by 3d to 5d postinjury.28 Almarghalani et al showed that M1 markers were significantly increased on 1d and 3d. In contrast, M2 markers were increased on 3d after cerebral hemorrhage, and the levels decreased from 7d and continued until 28d.29 Our study indicated that after stroke, pro-inflammatory factors reached peak time from 1d to 3d and remained elevated for at least 14d, while anti-inflammatory factors began to express from 1d and gradually increased over time. The differences may be due to varying degrees of damage to neurons and microglia in different brain regions after injury, as well as differences in individuals’ immune systems and metabolic states, which may lead to inconsistent expression of inflammatory factors. These above data further elucidate effects of Exos on stroke, but large-scale human clinical trials have not yet been conducted. A study on animal experiments showed that NSC-Exos can alleviate post-stroke neuroinflammation and promote regeneration of neural tissue.5 Another clinical trial demonstrated that mesenchymal stem cell-derived exosomes could be safely injected into patients without any serious adverse reactions or complications and also exhibited better results in terms of neurological function recovery.30
In conclusion, NSC-Exos as a novel cell therapy drug, although currently facing some challenges, still has very optimistic development prospects for treating stroke. Our next step is to investigate the exosome metabolism by which the specific components of NSC-Exos regulate microglial polarization and to explore therapeutic mechanisms for alleviating inflammation.
Conclusion
In the present study, we identified the potentials of NSC-Exos administration in vivo in improvement of neurobehavioral recovery, infarct volume, inflammation cytokines, and microglia to anti-inflammatory phenotype polarization. Accordingly, our data support that NSC-Exos is feasible to be considered as a therapeutic approach in cerebral ischemia.
Acknowledgments
This study was supported by the National Natural Science Foundation of China (81660243); the Natural Science Foundation Project of Guizhou Provincial Science and Technology Department (Qiankehe Foundation-ZK [2021,2023] General 415,323); and the National Natural Science Foundation of Guizhou Medical University (20NSP006).
Disclosure
The authors report no conflicts of interest in this work.
References
1. Akinyemi RO, Owolabi MO, Ihara M, et al. Stroke, cerebrovascular diseases and vascular cognitive impairment in Africa. Brain Res Bull. 2019;145:97–108. doi:10.1016/j.brainresbull
2. Congqin L, Ying X, Yuqian Z, et al. Neutrophil extracellular traps exacerbate ischemic brain damage. Mol Neurobiol. 2021;59(1):643–656. doi:10.1007/s12035-021-02635-z
3. Jurga AM, Paleczna M, Kuter KZ. Overview of general and discriminating markers of differential microglia phenotypes. Front Cell Neurosci. 2020;14:198–215. doi:10.3389/fncel.2020.00198
4. Han B, Jiang W, Cui P, et al. Microglial PGC-1α protects against ischemic brain injury by suppressing neuroinflammation. Genome Med. 2021;13(1):47–65. doi:10.1186/s13073-021-00863-5
5. Leng F, Edison P. Neuroinflammation and microglial activation in Alzheimer disease: where do we go from here? Nat Rev Neurol. 2020;17(3):157–172. doi:10.1038/s41582-020-00435-y
6. Qin C, Zhou LQ, Ma XT, et al. Dual functions of microglia in ischemic stroke. Neurosci Bull. 2019;35(5):921–933. doi:10.1007/s12264-019-00388-3
7. Zeng X, Geng W, Jia J, et al. Advances in stem cells transplantation for the therapy of Parkinson’s disease. Curr Stem Cell Res Ther. 2021;16(8):958–969. doi:10.2174/1574888X16666210309153949
8. Zhu JD, Wang JJ, Ge G, et al. Effects of Noggin-transfected neural stem cells on neural functional recovery and underlying mechanism in rats with cerebral ischemia reperfusion injury. J Stroke Cerebrovascular Dis. 2017;26(7):1547–1559. doi:10.1016/j.jstrokecerebrovasdis.2017.02.034
9. Hwang I, Hong S. Neural stem cells and its derivatives as a new material for melanin inhibition. Int J Mol Sci. 2017;19(1):36–53. doi:10.3390/ijms19010036
10. Yuluo R, Wei L, Jiaxing W, et al. Neural stem cell-derived small extracellular vesicles attenuate apoptosis and neuroinflammation after traumatic spinal cord injury by activating autophagy. Cell Death Dis. 2019;10(5):340–357. doi:10.1038/s41419-019-1571-8
11. Zhong D, Cao Y, Li CJ, et al. Neural stem cell-derived exosomes facilitate spinal cord functional recovery after injury by promoting angiogenesis. Exp Biol Med. 2020;245(1):54–65. doi:10.1177/1535370219895491
12. Vogel A, Upadhya R, Shetty AK. Neural stem cell derived extracellular vesicles: attributes and prospects for treating neurodegenerative disorders. EBioMedicine. 2018;38:273–282. doi:10.1016/j.ebiom.2018.11.026
13. Longa E, Weinstein PR, Carlson S, et al. Reversible middle cerebral Artery occlusion without craniectomy in rats. Stroke. 1989;20(1):84–91. doi:10.1161/01.str.20.1.84
14. Li S, Luo L, He Y, et al. Dental pulp stem cell-derived exosomes alleviate cerebral ischaemia-reperfusion injury through suppressing inflammatory response. Cell Prolif. 2021;54(8):e13093. doi:10.1111/cpr
15. Vorhees CV, Williams MT. Morris water maze: procedures for assessing spatial and related forms of learning and memory. Nat Protoc. 2006;1(2):848–858. doi:10.1038/nprot.2006.116
16. Jin Y, Barnett A, Zhang Y, et al. Poststroke sonic hedgehog agonist treatment improves functional recovery by enhancing neurogenesis and angiogenesis. Stroke. 2017;48(6):1636–1645. doi:10.1161/STROKEAHA.117.016650
17. Liu X, Zhang M, Liu H, et al. Bone marrow mesenchymal stem cell-derived exosomes attenuate cerebral ischemia-reperfusion injury-induced neuroinflammation and pyroptosis by modulating microglia M1/M2 phenotypes. Exp Neurol. 2021;341(28):113700. doi:10.1016/j.expneurol.2021.113700
18. Song Y, Li Z, He T, et al. M2 microglia-derived exosomes protect the mouse brain from ischemia-reperfusion injury via exosomal miR-124. Theranostics. 2019;9(10):2910–2923. doi:10.7150/thno.30879
19. Berchtold C, Priller J, Meisel C, Meisel A. Interaction of microglia with infiltrating immune cells in the different phases of stroke. Brain Pathol. 2020;30(6):1208–1218. doi:10.1111/bpa.12911
20. Jie T, Yu W, Sheng C, et al. Microglia polarization and endoplasmic reticulum stress in chronic social defeat stress induced depression mouse. Neurochem Res. 2018;43(3):985–994. doi:10.1007/s11064-018-2504-0
21. Qin C, Fan WH, Liu Q, et al. Fingolimod protects against ischemic white matter damage by modulating microglia toward M2 polarization via STAT3 pathway. Stroke. 2017;48(12):3336–3346. doi:10.1161/STROKEAHA.117.018505
22. Zhou L, Zhu H, Bai X, et al. Potential mechanisms and therapeutic targets of mesenchymal stem cell transplantation for ischemic stroke. Stem Cell Res Ther. 2022;13(1):195–209. doi:10.1186/s13287-022-02876-2
23. Long X, Yao X, Jiang Q, Yang Y, Zhang H. Astrocyte-derived exosomes enriched with miR-873a-5p inhibit neuroinflammation via microglia phenotype modulation after traumatic brain injury. J Neuroinflammation. 2020;17(1):89–103. doi:10.1186/s12974-020-01761-0
24. Halurkar MS, Wang J, Chen S, Bihl JC. EPC-EXs improve astrocyte survival and oxidative stress through different uptaking pathways in diabetic hypoxia condition. Stem Cell Res Ther. 2022;13(1):91–100. doi:10.1186/s13287-022-02766-7
25. Webb RL, Kaiser EE, Jurgielewicz BJ, et al. Human neural stem cell extracellular vesicles improve recovery in a porcine model of ischemic stroke. Stroke. 2018;49(5):1248–1256. doi:10.1161/STROKEAHA.117.020353
26. Zhu Z, Jia F, Ahmed W, et al. Neural stem cell-derived exosome as a nano-sized carrier for BDNF delivery to a rat model of ischemic stroke. Neural Regen Res. 2023;18(2):404–409. doi:10.4103/1673-5374.346466
27. Luo L, Liu M, Fan Y, et al. Intermittent theta-burst stimulation improves motor function by inhibiting neuronal pyroptosis and regulating microglial polarization via TLR4/NFκB/NLRP3 signaling pathway in cerebral ischemic mice. J Neuroinflammation. 2022;19(1):141–167. doi:10.1186/s12974-022-02501-2
28. Hu X, Li P, Guo Y, et al. Microglia/macrophage polarization dynamics reveal novel mechanism of injury expansion after focal cerebral ischemia. Stroke. 2012;43(11):3063–3070. doi:10.1161/strokeaha.112.659656
29. Almarghalani D, Sha X, Mrak R, Shah Z. Spatiotemporal cofilin signaling, microglial activation, neuroinflammation, and cognitive impairment following hemorrhagic brain injury. Cells. 2023;12(8):1153–1172. doi:10.3390/cells12081153
30. Dehghani L, Khojasteh A, Soleimani M, et al. Safety of intraparenchymal injection of allogenic placenta mesenchymal stem cells derived exosome in patients undergoing de compressive craniectomy following malignant middle cerebral artery infarct, A pilot randomized clinical trial. Int J Prev Med. 2022;13:7–14. doi:10.4103/ijpvm.ijpvm_441_21
© 2023 The Author(s). This work is published and licensed by Dove Medical Press Limited. The full terms of this license are available at https://www.dovepress.com/terms.php and incorporate the Creative Commons Attribution - Non Commercial (unported, v3.0) License.
By accessing the work you hereby accept the Terms. Non-commercial uses of the work are permitted without any further permission from Dove Medical Press Limited, provided the work is properly attributed. For permission for commercial use of this work, please see paragraphs 4.2 and 5 of our Terms.