Back to Journals » Journal of Inflammation Research » Volume 16

Manjari Medika Grape Seed Extract Protects Methotrexate-Induced Hepatic Inflammation: Involvement of NF-κB/NLRP3 and Nrf2/HO-1 Signaling System
Authors Manna K, Khan ZS, Saha M, Mishra S, Gaikwad N, Bhakta JN, Banerjee K, Das Saha K
Received 17 September 2021
Accepted for publication 20 August 2022
Published 7 February 2023 Volume 2023:16 Pages 467—492
DOI https://doi.org/10.2147/JIR.S338888
Checked for plagiarism Yes
Review by Single anonymous peer review
Peer reviewer comments 3
Editor who approved publication: Professor Ning Quan
Krishnendu Manna,1,* Zareen S Khan,2,* Moumita Saha,3,* Snehasis Mishra,3 Nilesh Gaikwad,4 Jatindra Nath Bhakta,1 Kaushik Banerjee,2 Krishna Das Saha3
1Department of Food & Nutrition, University of Kalyani, Nadia, West Bengal, India; 2National Referral Laboratory, ICAR-National Research Centre for Grapes, Pune, Maharashtra, 412307, India; 3Cancer Biology and Inflammatory Disorder Division, CSIR-Indian Institute of Chemical Biology, Kolkata, West Bengal, 700032, India; 4ICAR-National Research Centre on Pomegranate, Solapur, Maharashtra, 413255, India
*These authors contributed equally to this work
Correspondence: Krishna Das Saha, Cancer Biology and Inflammatory Disorder Division, CSIR-Indian Institute of Chemical Biology, 4, Raja S.C. Mullick Road, Kolkata, West Bengal, 700032, India, Tel +91 33 2499 5810, Fax +91 33 2473 5197, Email [email protected] Kaushik Banerjee, National Referral Laboratory, ICAR-National Research Centre for Grapes, Pune, Maharashtra, 412307, India, Email [email protected]
Objective: Grape Seed Extract is a natural source of various polyphenols, which have been shown to possess potent antioxidant and free radical-scavenging activities. The earlier studies have reported that grape seed extract exhibits broad-spectrum pharmacological activities. Therefore, studying the hepatoprotective effects and elucidation of mechanisms of action of the Indian Variety, Manjari Medika grape seed extract (GSE), may give an insight into therapeutic benefits. Methotrexate (MTX) is the first-line pharmacological therapy for different rheumatic diseases. The major adverse events such as hepatotoxicity are evident even in the low doses used for the treatment. The present study investigated the role of MTX on hepatic damage in murine liver and the plausible protective effects of the Indian grape variety, Manjari Medika grape seed extract, in ameliorating it.
Methods and Results: To assess the hepatological modulation, mice were divided into eight groups to investigate the ameliorative potential of this GSE (75 and 125 mg/kg) and correlate the experimental findings. The active components of the extract were assessed through UPLC-(ESI)-QToF-MS analysis. On the other hand, various biochemical and immunological indices were carried out to correlate the experimental data. The result demonstrated that the prophylactic administration of GSE reduced MTX-induced hepatic toxicity indices, which subsequently restored the hepatic morphological architecture. Moreover, the application of GSE in a dual dosage (75 and 125 mg/kg) suppressed MTX-induced reactive oxygen species generation, followed by lipid peroxidation and cellular nitrite formation. MTX-induced inflammasome activation through the redox-assisted cascade of TLR4/NF-κB signaling was further reduced by applying the GSE. The results showed that the activation of cytoprotective transcription factor Nrf2 enhanced the level of endogenous antioxidants. Furthermore, through the regulation of TLR4/NF-κB and Nrf2/HO-1 axis, this extract could reduce the MTX-mediated hepatic damage.
Conclusion: Our findings suggest that Manjari Medika seed extract could be used as a therapeutic agent to relieve the side effects of MTX and other hepatic disorders.
Keywords: hepatic fibrosis, Kupffer cells, Manjari Medika grape seed extract, proanthocyanidins, inflammasome, antioxidant response
Graphical Abstract:
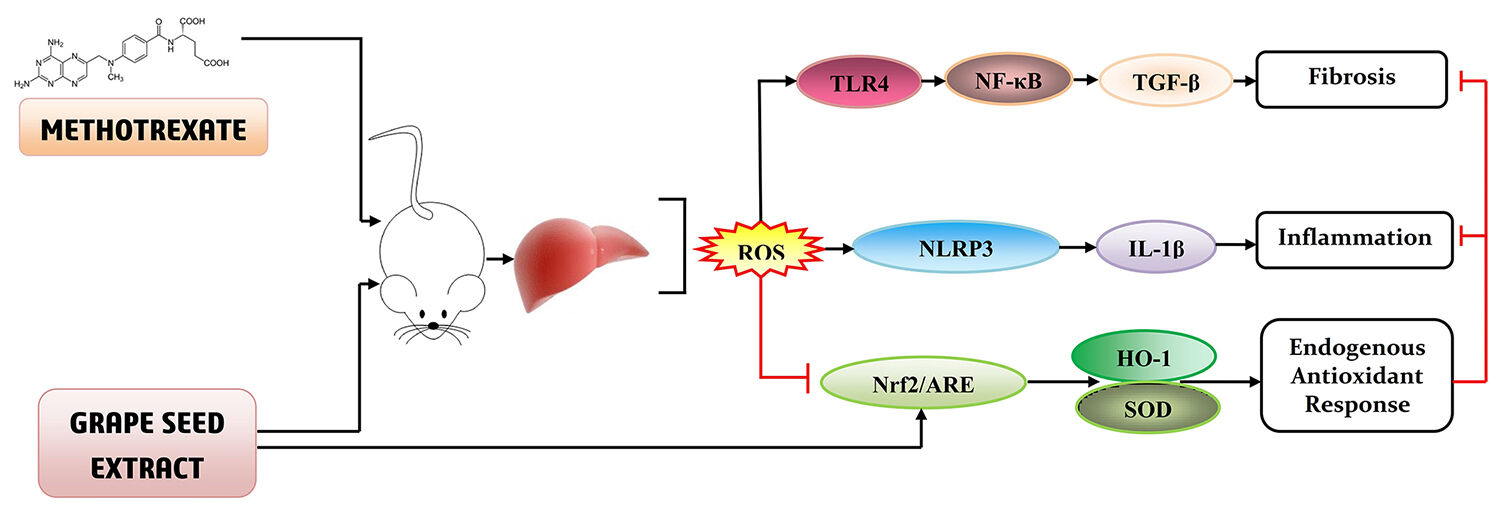
Introduction
Recently, drug-induced liver damage has been a significant concern for human health.1 Methotrexate (MTX), a folate antagonist, is widely used as a cytotoxic chemotherapeutic agent for treating breast, bone, lymph, head, and neck carcinoma.2,3 It is also used for managing rheumatism,4 psoriasis, and Crohn’s disease5 and is a much cheaper drug than the available therapeutics on the market. MTX is mentioned in the “World Health Organization’s List of Essential Medicines” as a therapeutic drug and should be accessible to every healthcare system.6 In order to determine the overall benefit of MTX therapy, numerous scientific reports and meta-analyses have revealed that it offers a better efficacy than readily available drugs.6 Being a well-tolerated immunosuppressant drug, MTX is associated with symptomatic detrimental events. So far, several adverse effects have been attributed to its chronic usage as well as high dosage intake. Researchers have identified a range of hepatic disorders associated with chronic MTX toxicity, including extensive collagen accumulation, fibrotic lesion, and inflammatory cell infiltration.7,8 Besides, interstitial lung disease is commonly observed in MTX-induced adverse events.
The nature of liver disease associated with the MTX treatment is complex. The exact pathophysiological reason for the MTX-induced hepatic disorder is unknown. It has been previously noted that MTX suppresses the dihydrofolate reductase, inhibiting the intercellular reservoir of folate needed for thymine synthesis.9 With the treatment of high dosages of MTX, abnormal T-cell apoptosis and enhanced cytokine production have been observed,10 suggesting the apparent effect on abnormal folate metabolism. On the other hand, an anti-inflammatory effect of MTX has been found in low dosages of treatment due to changes in adenosine signaling rather than folate metabolism.11 In the adenosine signaling system, the inhibitory effect on 5-aminoimidazole-4-carboxamide ribonucleotide formyltransferase (AICR TFase) enhances the extracellular adenosine formation, which promotes hepatic fibrosis.11 Earlier reports have demonstrated that the use of MTX enhanced hepatic transaminases at a chronically low dosage.12 The prevalence of significant hepatic fibrosis and steatosis was also observed in a patient taking MTX.13 A growing body of evidence suggests that chronic MTX treatment could eventually lead to hepatic disorder.13 The liver is a vital metabolic organ that consists of hepatic parenchyma and a large proportion of nonparenchymal cells (NPCs). These include the Kupffer cell, which is a dedicated hepatic resident macrophage and has a role in regulating drug-induced hepatotoxicity and in the initiation and progression of liver diseases.14 Kupffer cell comprises a significant population of inflammatory cells in the liver and plays a vital role in hepatic homeostasis. During chronic injury, a large population of extrahepatic inflammatory cells migrate towards the damage site and alter the liver’s immune cell population.15 Researchers have noted that several drugs activate Kupffer cells and induce the release of a plethora of pro-inflammatory cytokines during chronic and acute liver injury.16 The onset of inflammatory response plays an essential role in triggering the fibrosis as a resident and extrahepatic macrophage, facilitating the release of various pro-inflammatory mediators.17 These mediators are thought to create an environment that amplifies the fibrogenic response. This, in turn, induces hepatocyte proliferation and leads to preneoplastic transformations. It has also been associated with a significant change in extracellular matrix formation (ECM). Therefore, Kupffer cells are essential in maintaining the balance of hepatic milieu.18 The importance of the Kupffer cell is not only in the initiation of fibrogenic injury but also in the progression of fibrosis during the drug-induced hepatic disorder.18 Thus, targeting the Kupffer cell regulation might provide an opportunity to develop targeted therapeutics for drug-induced liver damage.
Fruit-based polyphenols are secondary metabolites that have been proven as potential therapeutic agents for the prevention of multiple diseases, including hepatic,19 cardiovascular,20 neurodegenerative,21 and metabolic diseases.22 Thus, they are gaining currency for demonstrating health benefits. Most plant polyphenols have several pharmacological effects on oxidative stress, metabolism, and inflammation, and these are the key pathological processes in the etiology of liver diseases.23 Furthermore, these features put polyphenols under the spotlight of investigation for the therapy of hepatic disease. A significant contribution of fruit-derived phytochemicals has created considerable attention towards managing different human ailments.24 These phytochemicals alone or in combination are believed to impart therapeutic effects and play a pivotal role in modulating disease states. It has shown effectiveness in treating various chronic diseases like viral,25 Alzheimer’s, inflammatory bowel diseases, arthritis, and common malignancies of the stomach, colon, skin, breast, and lung.26 Epidemiological and preclinical data depicted that phytochemicals delay the pathophysiological process of different diseases or act as chemopreventive agents by interacting with the molecular mechanism.27 According to many researchers, regular consumption of plant-based diet rich in vegetables, fruits, flowers, nuts, seeds containing polyphenols, terpenoids, and phytosterol exert their beneficial effect by regulating different cellular and molecular signaling pathways.24,28 Many studies demonstrated that phytochemicals are used in cancer therapeutics,29,30 which influence the targets and signaling pathways involved in proliferation, invasion, metastasis, and angiogenesis.31–33 Phytochemicals contribute to the modulating effect by altering the pro-survival signaling pathways as well as anti-apoptogenic pathways in cancer.34,35 The mechanistic pathways can influence DNA repair, cell cycle regulation, cell death, or apoptosis. Altered activation is involved in pleiotropic cytotoxic and cytoprotective responses, leading to the activation or inhibition of several signaling cascades. Phytochemicals can exert their effect by altering redox-sensitive transcription factor signaling like NF-κB, JAK/STAT, AP-1, p53.36 Besides, it can also mediate their anti-oxidative effect by triggering Nrf2/ARE/HO-1 signaling system.37 However, there is a significant translational gap in using these phytochemicals from bench to bedside.
Over the past decades, natural antioxidants such as vitamin C, vitamin E, and multiple polyphenols have been proven as the functional compounds in grapes that prevent free radical damage to cells.38 Polyphenols, an abundantly found class of bioactive component in grapes, have also drawn significant attention because they promote a wide range of health benefits. A grape seed extract has been reported as a curative agent for managing many diseases,39 which includes hepatic disorders. GSE is a rich source of flavonoids, including proanthocyanidin oligomers. It is known to exert cardioprotective, anti-diabetic, anti-obesogenic, anti-bacterial, anti-bacterial, and anti-mutagenic properties.40,41 Although grape seed proanthocyanidins (GSP) are known to demonstrate preventive and therapeutic properties against oxidative stress-associated cellular anomalies,38 its underlying mechanism remains elusive. So far, little efforts have been set forward to assess the hepatoprotective activity underlying the precise molecular mechanism of GSP on the modulation of redox-regulated transcription factor-triggered inflammasome activation in Kupffer cells.
Manjari Medika (MM) is an Indian grape variety, which is lately developed by hybridizing Pusa Navrang (a seeded juice variety) with Flame Seedless (a red-colored table grape variety) cultivars.42 Previous research revealed that MM seed is not only a rich source of anthocyanins and other flavonoids43 but also has a high level of Vitamin E (1.15–1.35 g/kg). Although the phytochemical profile of GSE indicates its role as a hepatoprotective therapeutics,44 there is no experimental or clinical data to understand whether this variety’s seed extract can prevent hepatocellular toxicity induced by MTX. The above-stated gap triggered our current interest in evaluating the protective role of GSE against MTX-induced hepatic damage. A particular emphasis was placed on assessing the effect of GSE on Kupffer cell function in regulating inflammation and antioxidant balance.
Materials and Methods
Chemicals
The pure and analytical grade chemicals were purchased from Merck Millipore (Billerica, Massachusetts, USA). The analytical standards for high-performance liquid chromatography (HPLC) were procured from Sigma-Aldrich Corporation (St Louis, Missouri, USA). The other reagents (analytical grade) were obtained from Sisco Research Laboratories (Mumbai, India). All antibodies were bought from Cell Signaling Technology (Danvers, MA, USA).
Plant Material Collection and Preparation of Grape Seed Extract
MM grape berries were harvested from the vineyards of Indian Council of Agriculture Research-National Research Centre for Grapes (ICAR-NRCG), Pune (altitude: 559 m above the mean sea level, latitude: 18.32°N, longitude: 7.51°E) at horticultural maturity [Brix value of total soluble solids = 20–24, pH = 3.58, total acidity = ~1.7 g/L, volatile acidity = ~0.04 g/L]. Following the extraction of juice, the seeds (35–40 g seed/kg of fresh grape) were recovered from pomace, washed with water, and dried in a vacuum drier (at <60°C) to a moisture content of ~7–10% (by weight). These were further homogenized to a fine powder. Using 50% ethanol in a ratio of 1:10 (seed: solvent), the seeds were extracted and then subjected to liquid chromatography-mass spectrometry (LC-MS) analysis by a previously reported method.45
LC-MS [UPLC-(ESI)-QToF-MS] Conditions
An Acquity Ultrahigh Performance Liquid Chromatography (UHPLC), coupled to a quadrupole-time of flight mass spectrometer (QToF-MS, Synapt G2 HDMS, Waters Corporation, Manchester, U.K.), was used for the screening of phytochemicals. The instrument was operated with electrospray ionization (ESI) and controlled by MassLynx 4.1 software. The mass resolution was set at 20,000. The high-resolution accurate mass analysis quickly swapped the entire scan acquisition at 4 V to 10–60 V in a single L.C. run. An Acquity UPLC BEH C18 column (2.1×100 mm, 1.8 μm, Waters India Pvt. Ltd., Bengaluru) was used for chromatographic separation. The mobile phase comprised A: methanol: water (10:90, v/v) and B: methanol: water (90:10, v/v), each containing 0.1% formic acid. The UHPLC flow rate was maintained at 0.4 mL/min. The gradient program started with 90% A (0–0.5 min), reduced to 50% A (4.5 min), further reduced to 2% A (4.5–8 min), and maintained at 2% A (8–11 min), enhanced to 90% A (11–11.5 min) and maintained at 90% A for another 3 min (12–15 min).46 The LC-MS data was processed using UNIFI software (Version 1.7, Waters Corporation, Milford, MA, USA). The mass error limit was set at 5 ppm to ensure enough accuracy in tentative identifications. The relative concentrations of the identified compounds were estimated against the certified reference standards of quercetin (for non-anthocyanins) (Sigma-Aldrich, St. Louis, Missouri, USA) and pelargonidin-3-O-glucoside (for anthocyanin derivatives) (Sigma-Aldrich, St. Louis, Missouri, USA).46
Animals
Adult male BALB/c mice (~8 to 10 weeks old, with initial body weights of 20–22 g) were procured from the inbred institutional facility. The animals were kept under standard laboratory conditions (at 21 ± 2°C with a relative humidity of 55%). Throughout this study, the 12-hours:12-hours light: dark cycle was maintained. The animals were given standard rat/mice pellets and tap water ad libitum. All experimental protocols were performed as per the guidelines of the Institutional Animal Ethics Committee, CSIR-Indian Institute of Chemical Biology (CSIR-IICB), and approved by the Committee for the Purpose of Control and Supervision of Experiments on Animals, Ministry of Environment, Forests and Climate Change, The Government of India (Approval No. IICB/AEC/Meeting/Feb/2018/1).
Experimental Design
The design of the overall experimentation is illustrated in Figure 1. Male mice were randomly divided into eight groups, each consisting of five mice. According to the CPCSEA norms, to reduce the number of animal usage in single experimentation, two sets of preliminary experiments were done previously using six animals in a group before the final study.
Group I: Mice (control) were kept at an ambient temperature of 24 ± 2°C with a relative humidity of 60–70%.
Group II: Mice received a single intraperitoneal (I.P) injection of MTX at a dose of 20 mg/kg body weight on the 7th day of the experiment.
Group III–IV: Mice were treated with a single intraperitoneal (I.P) injection of MTX at a dose of 20 mg/kg body weight on the 7th day along with the pre- and post-application of GSE, orally fed in a dual dose (75 and 125 mg/kg) until the termination of the experiment. The final dose was chosen based on the preliminary experimentation. The chosen dosage was clinically relevant and was based on similar published reports earlier.47,48
Group V–VIII: The animals were respectively administrated with N-Acetylcysteine (NAC) (300 mg/kg body weight, i.p),49 0.2% Gadolinium chloride (GdCl3) (20 mg/kg body weight, i.v.),50 glyburide (132.5 mg/kg, i.p)51 (Sigma-Aldrich, St. Louis, Missouri, USA) and Toll-like receptor 4 (TLR4) inhibitor (TAK-242) (3 mg/kg, i.v)52 (Calbiochem, St. Louis, Missouri, USA) after the MTX-treatment for three alternate days (8th, 10th, and 12th).
Food was withdrawn 24 hours before the final day of the experiment (ie, the 14th day), and each mouse was weighed before decapitation. Their blood samples were drawn through the retro-orbital puncture, and the liver tissues were rapidly excised and weighed. The euthanasia of all animals was carried out according to the rules stipulated by CPCSEA, Government of India. The procedure was carried out quickly and painlessly in an atmosphere free from fear or anxiety. For a euthanasia method of an experimental animal, an initial depressive action on the central nervous system was made for immediate insensitivity to pain.
Each animal’s longitudinal section of the right liver was used for histopathological and immunohistochemical examinations. The remainder of liver tissue was collected to assess the other biochemical and molecular aspects. Single-cell isolation was done from the freshly dismembered liver and subjected to the flow cytometric evaluation.
Histopathological Examination
Treated tissues were embedded in paraffin and cut into thin sections (1 mm3) through microtome, followed by hematoxylin and eosin staining. All the stained slides were then observed under light microscopy. Histological alterations were assessed using a previously established histopathological scoring protocol with slight modifications.53 Six visual fields were randomly selected for each histopathological section, visualized under a high-power microscope (magnification, 20×), and then blindly scored by a pathologist. The inflammation score was presented as a sum of the severity of inflammation, an extension of inflammation (none, 0; slight, 1; moderate, 2; and severe, 3), and the necrosis was scored as none, 0; focal confluent necrosis, 1; Zone 3 necrosis in some areas, 2; Zone 3 necrosis in most areas, 3; Zone 3 necrosis+occasional portal-central bridging, 4; Zone 3 necrosis+multiple P-C bridging, 5; Panacinar or multiacinar necrosis, 6.
Isolation of Hepatocyte and Kupffer Cell
Briefly, the inferior vena cava was cannulated following a midline incision, and the portal vein was severed. Immediately, the liver was perfused in situ with HBSS (without Ca2+ and Mg2+), followed by another perfusion with Hanks’ Balanced Salt Solution (HBSS) containing Type IV collagenase (0.025%) and calcium chloride (CaCl2) (100 nM) at a rate of 15 mL/min while maintaining the temperature at 37°C. Afterward, the liver was removed and rinsed with fresh HBSS. It was minced in a Petri dish containing HBSS with collagenase (Type IV) (Sigma-Aldrich, St. Louis, Missouri, USA) and incubated for 20 min at 37°C for further segregation of the cells. After that, the resultant cell suspension was passed through a sterile cell strainer (70 μm) into a cold Dulbecco’s Modified Eagle Medium (DMEM) (Thermo Fisher Scientific, Waltham, Massachusetts, USA) which contained 10% heat-inactivated fetal bovine serum (FBS). This mixture was centrifuged at 50×g for 2 min at 4°C to sediment the hepatocytes. The remaining cells in the supernatant were centrifuged and used for another density gradient centrifugation (1000×g for 10 min at 4°C) using Percoll (Sigma-Aldrich, St. Louis, Missouri, USA). While the Kupffer cell fraction cell pellets were resuspended in the fresh HBSS, the remaining supernatant was discarded. The Kupffer cells were allowed to adhere to the bottom of the plastic culture dish for 4 hours. The cells that did not settle down were removed by mild washing.54 The cell viability was determined by using a Trypan blue (Thermo Fisher Scientific, Waltham, Massachusetts, USA) exclusion assay. Throughout, the isolated hepatocyte and Kupffer cells were used.
Determination of Hepatic Intracellular Reactive Oxygen Species (iROS) and Cellular Nitrite
The level of iROS and nitric oxide (NO) was measured using the oxidation sensor dye, 2′,7′-dichlorofluorescein diacetate (H2DCFDA) (Invitrogen, Waltham, Massachusetts, USA) and 4-amino-5-(methylamino)-2′,7′-difluorofluorescein diacetate (DAF-FM DA) (Invitrogen, Waltham, Massachusetts, USA), wherein an increase in green fluorescence intensity was used to quantify the generation of intracellular ROS and NO with regard to untreated control. The harvested hepatic cells (2×106) were resuspended in the medium containing H2DCFDA and DAF-FM DA and later incubated at 37°C for 30 min. The resultant fluorescence was acquired by BD FACSAria IIITM flow cytometer (Becton Dickinson, Franklin Lakes, NJ, USA) coupled with an argon laser at 488 nm.55
Determination of Lipid Peroxidation (LPO)
According to the manufacturer’s instruction (ThermoFisher Scientific, Waltham, Massachusetts, USA), lipid peroxidation was detected through lipophilic probe BODIPY using flow cytometry.
Preparation of Tissue Homogenate
The hepatic tissues from the control and experimental groups of mice were excised, rinsed with ice-cold saline water, and homogenized in tris(hydroxymethyl)aminomethane hydrochloride (Tris-HCl) buffer (100 mM, pH 7.4) using a Teflon homogenizer. This mixture was centrifuged at 12,000×g for 30 min at 4°C. For biochemical evaluation, the supernatant was pooled and estimated. The protein content of the tissue homogenate was also estimated.56
Estimation of Hepatic Toxicity Marker
The hepatic toxicity indices, alkaline phosphatase (ALP), alanine aminotransferase (ALT), aspartate aminotransferase (AST), and lactate dehydrogenase (LDH) were determined using the commercially available kits (Arkray, Kyoto, Japan) and performed following the manufacturer’s protocols.
Determination of Protein Expression Using Flow Cytometry
The quantification of intercellular protein expression was performed using flow cytometry through indirect immunofluorescence techniques. Briefly, the isolated hepatocyte cells/Kupffer cells were fixed in paraformaldehyde (4%) in phosphate buffer saline (PBS) (pH 7.4) for 20 min at room temperature and permeabilized in Triton X-100 (0.1%) in PBS (with FBS, 0.1%) for 5 min. Following that, the permeabilized single cells were incubated with the primary antibody (1:200) against super oxide dismutase (SOD), catalase, alpha-1 antitrypsin (α-1AT), CD11b, NLR family pyrin domain containing 3 (NLRP3), Interleukin 1 beta (IL-1β), caspase-1, TLR4, nuclear factor kappa-light-chain-enhancer of activated B cells- p65 subunit (NF-κB-p65), and Nrf2 for 120 min at 4°C. Afterward, the cells were washed with PBS and incubated for 30 min at 4°C in the presence of fluorescein isothiocyanates (FITC)/Alexa Fluor 488/Phycoerythrin (PE)/Alexa Fluor 647-conjugated anti-mouse/rabbit IgG antibody. The stained cells were acquired by using BD FACSAria IIITM (Becton Dickinson, Franklin Lakes, NJ, USA). The resultant fluorescence intensity was analyzed through Flow Jo software (V10). Similar to our earlier report,57 10,000 cells were analyzed in each experiment.
Determination of Protein Expression Using Immunofluorescence
The unstained hepatic section (6 µm of thickness) of all experimental groups was analyzed through indirect immunofluorescence assay.57 Briefly, the sections were deparaffinized and hydrated in graded ethanol, followed by blocking (0.3% hydrogen peroxide) for 20 min to mask the endogenous peroxidase activity. A microwave oven was used to retrieve the antigen using citrate buffer (10 mM, pH 6.0) at 95–98°C for 15 min. At 37°C, these sections were further blocked with bovine serum albumin (BSA) (5%) for 30 min. Later, they were incubated at 4°C overnight with the primary antibody against transforming growth factor-β (TGF-β), alpha-smooth muscle actin (α-SMA), nuclear factor erythroid 2–related factor 2 (Nrf2), and heme oxygenase-1 (HO-1) (Dilution: 1:250). After washing with PBS, these were incubated with anti-rabbit/mice-FITC/PE secondary antibody for 1 hour each at 37°C, followed by nuclear staining with 4′,6-diamidino-2-phenylindole (DAPI) (Santa Cruz Biotechnology, Dallas, Texas, United States) for 10 min. The images were observed in Olympus FV1000 MPE SIM Laser Scanning confocal microscope (Olympus, Tokyo, Japan).57,58 The resultant intensity was analyzed using ImageJ software (NIH, Maryland, United States).
Preparation of the Cytosolic and Nuclear Fraction
The extraction of cytosolic and nuclear fractions was done by an earlier published method.55 Briefly, hepatic tissue was suspended in 100 μL hypotonic buffer (10 mM KCl, 1.5 mM MgCl2, 10 mM HEPES, 1 mM dithiothreitol, pH-7.9) containing protease inhibitor cocktail and was lysed by homogenization followed by centrifugation at 10,000g for 20 min. The resultant supernatant was then collected as a cytosolic fraction. The nuclear pellet was re-suspended in 50 μL nuclear extraction buffer (1.5 mM MgCl2, 0.2 mM EDTA, 0.42 M NaCl, 20 mM HEPES, 1 mM dithiothreitol, 25% (v/v) glycerol) containing the protease inhibitor cocktail, homogenized and incubated overnight at 4°C. Finally, the lysate was centrifuged at 20,000g for 5 min to collect supernatant as nuclear fraction.55
Immunoblotting
The earlier published protocol was adopted and slightly modified for the immunoblotting method.55,59 Briefly, 50 μg of nuclear and cytosolic protein was separated through SDS polyacrylamide gel (12.5%) and then transferred onto a PVDF membrane (Bio-Rad, Munich, Germany). The membranes were blocked with non-fat milk solution (5%) overnight and incubated with anti-rabbit/mouse polyclonal/monoclonal antibody (NLRP3, ASC1, Caspase-1, IL-1β, TLR4, and β-Actin) (1:1000) for 3 hours, followed by incubation with a Horseradish peroxidase (HRP)-conjugated antibody (1:2500) for 2 hours. After washing, the immune complex on the membrane was developed using an enhanced chemiluminescence detection system followed by densitometric analysis through ImageJ software (NIH, Bethesda, MD, USA).
Enzyme-Linked Immunosorbent Assay
ELISA was performed to evaluate the specific protein expression in tissue homogenates. Treated hepatic tissue samples were analyzed using the specific ELISA kits for Nrf2 (Novus Biologicals, CO, USA), NF-κB-p65 (RayBiotech, GA, USA), and HO-1 (Abcam, Cambridge, USA) according to the manufacturer instruction.
Statistical Analysis
All the values in the figures are expressed as mean values with their standard errors (Mean ± SEM) of n observations, where n (n = 6) represents the number of animals studied. Statistical software, OriginPro version 8.0 (OriginLab Corporation, Northampton, USA), was used for the data analysis. The statistical significance was evaluated using a one-way ANOVA through the Tukey method with the post-hoc test. The critical significance level was set at p<0.05.
Results and Discussion
Characterization of GSE
In this study, a total of fifteen phenolic compounds were putatively identified. These included pelargonidin-3-O-coumaroylglucoside, catechin (L-), malvidin-3-O-glucoside, procyanidin trimer EEC, malvidin-3-O-arabinoside, dihydrocaffeic acid-3-O-glucuronide, malvidin-3-O-coumaroylglucoside, catechin gallate, malvidin-3-O-acetylglucoside, peonidin-3-O-(6-p-coumaroylglucoside), enterodiol, rosmadial and quercetin-3-O-glucuronide (Table 1). Amongst the above compounds, pelargonidin-3-O-coumaroylglucoside, catechin (L), malvidin-3-O-glucoside, procyanidin trimer EEC and malvidin-3-O-arabinoside were the most prominent ones, having the characteristic pseudomolecular masses at 579.15, 291.08, 493.13, 867.21 and 463.12 Da, respectively (Table 1). The total phenol content in GSE was 2041 mg/L of gallic acid (G.A.) equivalent (mg/L), displaying a total antioxidant activity of about 86.85%. The presence of these polyphenolic compounds suggested the basis of antioxidant and hepatoprotective properties of GSE. Our in-house experimental results indicated a reduction in oxidative stress as a result of the GSE treatment. In the current study, the widely accepted mechanism behind hepatoprotection involved the amelioration of MTX-induced hepatic anomalies due to the free radical scavenging activity of GSE.60 This might relate to the high level of natural antioxidants in GSE. It contained a large variety of bioactive polyphenols, including acylated anthocyanins and other flavonoids. Earlier reports demonstrated a crucial role of dietary polyphenols supplementation in the modulation of pro-inflammation.61 Primarily, it could inhibit the expression of inducible nitric oxide synthase (iNOS), cyclooxygenase-2 (COX-2), NF-κB, and mitogen-activated protein kinases (MAPKs).
![]() |
Table 1 Characterization of Phenolic Compounds of GSE Using LC-ESI/MS Analysis |
GSE Suppressed MTX-Induced Hepatic Toxicity Marker
As mentioned earlier, MTX is an antineoplastic and immunosuppressive agent and is widely used for the treatment of carcinoma and inflammatory diseases.62 The prolonged therapy of MTX could be lethal as it affects liver enzymes and has been associated with the development of hepatic fibrosis, leading to cirrhosis.63 The enzymatic activity of hepatic function indicators, namely ALP, ALT, AST, and LDH, was measured to evaluate the modulatory potential of GSE on MTX-induced hepatic anomalies. As depicted in Figure 2A–D, the augmented activity of ALP, AST, ALT, and LDH was observed at 3.06-fold, 7.17-fold, and 4.95-fold and 6.66-fold, respectively, compared to the control. The treatment with two different doses of GSE showed a prominent decrease in the activity of ALP, ALT, AST, and LDH. The above readings indicate that GSE could alleviate MTX-induced hepatic injury.
GSE Reversed MTX-Induced Hepatic Architecture
To confirm the MTX-induced hepatic damage as well as to determine the ameliorative potential of GSE underlying its hepatoprotection, histopathological analysis was carried out. The control group showed a typical cellular architecture with distinct hepatic cells, sinusoidal space, and the central vein. On the other hand, the regular arrangements of hepatic cells with sinusoidal space were disturbed in the treated population. In addition, hepatic anomalies, including excessive vacuolization around the central vein, fatty degeneration, infiltration of inflammatory cells, and pyknotic nuclei, were also evident. These results could be substantiated by the earlier reports.63 In contrast, the application of GSE remarkably restored the pathological features of the MTX-treated condition, confirming its hepatoprotective efficacy. This might be due to the presence of several bioactive phenolic components in the seed of the MM grape (Figure 2E). The relative quantitative scoring analysis also validated the histological micrographs (Figure 2F and G).
GSE Suppressed MTX-Induced Intracellular Reactive Oxygen Species (iROS) in Hepatic Cells
iROS is a crucial player in drug-induced hepatic toxicity, which by triggering the downstream mediators, leads to hepatic fibrosis.64 To investigate the pathophysiological role of iROS in MTX-treated hepatic toxicity and its modulation by GSE, a flow cytometric approach was undertaken using the cell-permeant specific indicator, H2DCFDA. As shown in the flow cytometric analysis (Figure 3A), the relative dichlorofluorescein (DCF) fluorescence in terms of DCF positive (+Ve) cells was significantly increased upon MTX treatment (95.6%) with respect to the control hepatic cells (31.7%). The relative DCF fluorescence represented the relative amount of iROS present in the cellular environment. The figure also depicts that a higher percentage of DCF +Ve hepatic cells in MTX-treated condition was reduced with GSE treatment in dual doses (85.2% for 75 mg/kg and 71.7% for 125 mg/kg). On the other hand, the administration of NAC combined with MTX markedly diminished the DCF fluorescence (62.7%). Also, this combination lowered the hepatic ALP, AST, ALT, and LDH levels, indicating the probable involvement of ROS in MTX-mediated hepatic damage.65
GSE Prevented MTX-Induced Hepatic Lipid Peroxidation
Numerous reports have demonstrated that iROS is involved in the reaction with polyunsaturated fatty acids of the cellular membrane and can trigger LPO.64 The end product of LPO is considered the second messenger of oxidative stress, which was thought to be the primary factor for drug-induced hepatic toxicity.66 To investigate the protective efficacy of GSE on MTX-induced hepatic toxicity, an assessment of LPO was carried out using the specific sensor, BODIPY, following the flow cytometric approach (Figure 3B). As seen in the flow cytometry analysis, when compared with the control cells which were isolated from liver after completion of the experiment, BODIPY +Ve population was markedly increased with MTX-treatment. Contrastingly, a remarkable inhibition in LPO in terms of lowered BODIPY +Ve cells was evident with the application of GSE in a dual dosage (75 and 125 mg/kg). In contrast, the animals treated with NAC abolished the MTX-mediated hepatic LPO. It indicated the ROS-guided activation of lipid peroxides in MTX-treated conditions and the mechanism of GSE-directed inhibition of lipid peroxide formation, followed by hepatic damage.
GSE Reduced MTX-Induced Cellular Nitrite Concentration
The cellular nitrite concentration is one of the potential mediators of hepatic inflammation as well as fibrotic lesion.67 In this study, cellular nitrite was detected using a specific indicator, DAF-FM, to investigate the role of cellular nitrite in MTX-induced hepatic damage underlying its modulation by GSE. As evident in Figure 3C, MTX-treatment significantly increased the DAF-FM fluorescence in terms of the DAF-FM +Ve population amongst the isolated hepatic cells. At the same time, a gradual decline in DAF-FM +Ve population was observed upon the treatment of GSE (75 and 125 mg/kg). Earlier reports demonstrated that the application of NAC with MTX-treatment could suppress hepatic injury by reducing the ROS and RNS. A similar observation was reflected as GSE could modulate MTX-induced hepatic inflammation by scavenging ROS and RNS.68
GSE Restored MTX-Induced Endogenous Antioxidant Depletion
The endogenous antioxidants, both enzymatic and non-enzymatic, are involved in the regulation of homeostatic balance during oxidative condition.67 Free radicals can be produced by the oxidative metabolism of chemotherapeutic drugs and are often responsible for developing hepatic disorders.69 The endogenous antioxidant system detoxifies the free radicals and stabilizes the adaptive response that maintains the tissue redox balance.69 The expression pattern of enzymatic antioxidant SOD and catalase was assessed through flow cytometry (Figure 3D–G) to determine the effect of GSE on the endogenous antioxidant system. The result showed that MTX-treatment significantly (p < 0.05) downregulated SOD (0.34-fold) and catalase (0.53-fold) expressions compared to the control hepatic cells, as observed from the relative fluorescence intensity of FITC (Figure 3E and G). Contrastingly, when GSE was applied at a dosage of 75 and 125 mg/kg, it distinctly (p < 0.05) augmented (0.37 and 0.74-fold, respectively, for SOD and 0.59 and 0.73-fold, respectively, for catalase) the expression of the antioxidant enzymes over the MTX-treated condition. As evident in Figure 3D–G, NAC treatment enhanced the SOD and catalase expressions over the MTX-induced condition. This also indicated a possible role of GSE in enhancing the endogenous antioxidant enzyme. A comparative assessment of two varieties of GSE (MM-GSE and CS-GSE) on the enhancement of endogenous antioxidant enzymes was also checked in order to determine the potent antioxidant efficacy of MM-GSE (Figure S1).
GSE Suppressed MTX-Induced Inflammasome Activation
Inflammation is one of the critical factors in drug-induced liver damage.70 A growing body of evidence has suggested that Kupffer cells, which represent 80–90% of the tissue-resident macrophages, are concerned with the pathogenesis of liver diseases (eg, non-alcoholic steatohepatitis, alcoholic liver disease, chemotherapeutic drug-induced liver fibrosis, etc.).71 Hepatic inflammation is guided by a group of multiprotein oligomers (inflammasome) responsible for regulating meta-inflammatory responses. It was shown that NLRP3 [a preeminent member of the NOD-like receptor (NLR) subfamily of pattern recognition receptor (PRR, an adaptor molecule)] and “apoptosis-associated speck-like protein containing a caspase recruitment domain” (ASC) PYCARD form a Caspase-1 activating complex, which is known as NLRP inflammasome.72 Previous reports suggested that Kupffer cells are the primary origin of NLRP3 activation, which triggers the release of pro-inflammatory cytokines IL-1β in the liver.73 The mode of inflammasome activation is a multilayered process: it begins with the activation of the critical redox-regulated transcription factor, NF-κB, followed by the up-regulation of NLRP3 and pro-IL-1β expression. At the final step of this process, the effector Caspase-1 cleaves the pro-IL-1β form IL-1β, resulting in the development of the pro-inflammatory condition.74 To investigate the MTX-induced activation of the inflammasome and its modulation by GSE, the expression pattern of NLRP3, Caspase-1 and IL-1β was checked through flow cytometry (Figure 4B–D). According to the phenotype and function, Kupffer cells are classified into two major subsets: CD11b+ and CD68+. The CD11b+ subset mediates inflammasome activation, which is the leading producer of T helper 1 (Th1) cytokines.75 The characterization of Kupffer cells was done using a specific marker, CD11b. Figure 4A demonstrates the presence of CD11b +Ve Kupffer cells was further increased (11.5%) with the treatment of MTX. On the other hand, the resultant cell population had no expression of α-1 AT (0.039%), which is a specific marker for hepatocyte population. Interestingly, with the application of GSE at the rate of 75 and 125 mg/kg, the increased CD11b +Ve cells were strikingly decreased (9.75% and 7.86% respectively), when compared with the MTX-treated condition. The results also showed that MTX-treatment up-regulated the NLRP3 (24.7%), IL-1β (83.7%) and caspase-1 (9.23%) expression over the control Kupffer cells. Moreover, with the approach of a dual dose, a notable suppression in the expression of NLRP3 (17.6% and 14.1%, at 75 and 125 mg/kg respectively), IL-1β (33.2% and 24.6% respectively) and caspase-1 (7.58% and 5.52% respectively) was observed. The treatment with GdCl3 [a known inhibitor of macrophage population as well as glyburide (a specific inhibitor of NLRP3)] significantly reduced the expression of CD11b +Ve population (4.29% and 5.50% respectively) along with NLRP3 (14.8% and 16.5% respectively), IL-1β (15.2% and 20.9% respectively) and caspase-1 (6.44% and 6.82% respectively) (Figure 4B–D). These suggest that GSE could modulate the activated Kupffer cell population in MTX-treated condition, following the inflammasome activation.
To further confirm the involvement of the NLRP3 inflammasome pathway, immunoblotting was also carried out. As seen in Figure 4E and F, NLRP3, ASC1, caspase 1, and IL-1β expression were markedly (p < 0.05) enhanced in the MTX-treated hepatic tissue. Contrastingly, a gradual downregulation was also observed while treated with GSE (75 and 125 mg/kg) in MTX-treated conditions. On the other hand, GdCl3 treatment lowered the NLRP3, ASC1, caspase 1, and IL-1β expression as GdCl3 inhibited the Kupffer cell population significantly. Glyburide also suppressed inflammasome components, suggesting the pivotal role of the NLRP3 pathway in MTX treatment. Moreover, the above data confirmed the underlying anti-inflammatory mechanism of GSE by inhibiting the NLRP3 inflammasome.
GSE Suppressed TLR4-Mediated NF-κB Activation in Kupffer Cells
A recent report has demonstrated that “TLR4-guided NF-κB activation” is an essential regulator of drug-induced hepatic fibrosis and is associated with inflammation.76 NF-κB, one of the vital redox-sensitive transcription factors, is involved in the transcriptional regulation of a plethora of genes related to pro-inflammation.76 Similarly, this study explored the role of GSE in the modulation of the MTX-induced TLR4/NF-κB axis and observed that it could reduce TLR4 +Ve (11.8% and 7.80% respectively) as well as NF-κB (p65) +Ve (10.7% and 6.67% respectively) population. However, upon this treatment with MTX, they were increased (14.6% for TLR4 and 13.3% for NF-κB). On the other hand, TAK-242, a specific inhibitor of TLR4, remarkably diminished the TLR4 (3.61%) as well as NF-κB (2.41%) expressions in MTX-treated conditions, suggesting a direct correlation between TLR4 and NF-κB activation in drug-induced hepatic damage. In addition, TAK-242 restored the histopathological features (Figure 5A and B).
Immunoblotting and ELISA were also done in order to confirm the findings. MTX treatment significantly (p < 0.05) increased the expression of TLR4 and p65. However, GSE in dual dosage treatment (75 and 125 mg/kg) gradually decreased (p < 0.05) the expression. In line with the observation of TAK-242 treatment, it was evident that GSE modulated NF-κB-guided inflammatory signaling through a TLR4-dependant way (Figure 5D–F). From the above result, it can be indicated that GSE polyphenols could block TLR4 activation and subsequently NF-κB and restore the hepatic physiology and associated inflammation.
GSE Activated Nrf2-Guided Antioxidant Response
As mentioned previously, polyphenols enhance antioxidant response through the upregulation of cytoprotective transcription factor Nrf2.77 To check the variation of Nrf2 expression upon the treatment of two varieties of GSE (MM-GSE and CS-GSE), a preliminary experimentation was done using ELISA. The data is supplied in the Supplementary Materials, showing the efficacy of MM-GSE on Nrf2 expression over CS-GSE (Figure S2). Nrf2 is an important transcription factor that regulates most of the cytoprotective genes. Earlier reports suggested that augmentation of Nrf2 can block the activation of NF-κB.78 As shown in Figure 5B, GSE (75 and 125 mg/kg) significantly enhanced the Nrf2 expression (8.81% and 13.4%, respectively) while impeding the NF-κB activation in MTX-treated condition (10.6%).
The immunofluorescence analysis was also carried out to confirm the GSE-guided Nrf2 expression further. Figure 5C depicts that MTX-treatment considerably suppressed the Nrf2 expression as well as cytoprotective protein, HO-1. Interestingly, the application of GSE at a dual dose (75 and 125 mg/kg) could enhance both Nrf2 and HO-1 expressions, as observed from the relative fluorescence intensity. When treated with sulforaphane (a specific activator of Nrf2), a drastic increase in the expression of Nrf2 and HO-1 was recorded over the MTX treatment. On the other hand, ELISA study also supported the observation. GSE in dual dosage (75 and 125 mg/kg) enhanced (p < 0.05) the nuclear expression of Nrf2 as well as HO-1 in MTX-treated condition, indicating the plausible correlation of Nrf2 nuclear translocation and antioxidant response (Figure 5G and H). These data also suggested that the GSE polyphenols might have blocked NF-κB through the activation of Nrf2.
GSE Prevented MTX-Induced Hepatic Fibrosis
Liver fibrosis results from excessive accumulation of ECM proteins and pro-fibrogenic factors.79 Among many inflammatory cytokines involved in liver fibrosis, TGF-β emerges to be the essential one.80 The regulation of TGF-β is assisted by the NF-κB signaling system. Researchers have suggested that “α-SMA positive hepatic stellate cell” is responsible for the accumulation of fibrotic tissue and hepatic fibrosis.81 The immunofluorescence assay was carried out to evaluate the expression of TGF-β as well as α-SMA in hepatic tissue (Figure 6). ELISA assay was also adopted in order to assess the other key fibrogenic factors (Fibronectin, Type-I Collagen, and total SMAD3). Upon the treatment of MTX, the augmentation of TGF-β expression along with α-SMA was evident, and this was gradually diminished with the application of GSE (at the doses of 75 and 125 mg/kg). A combination of sulforaphane and MTX noticeably diminished TGF-β expression, which indicates Nrf2-guided inhibition of NF-κB/TGF-β activation. The other fibrogenic factor also (Fibronectin, Type-I Collagen, and total SMAD3) modulated upon the treatment of MTX and GSE (75 and 125 mg/kg) (Figure S3). This, in turn, suggests that GSE might have played an essential role in reducing the TGF-β expression by modulating the cross-talk machinery of Nrf2 and NF-κB.
Discussion
Drug-induced liver injury is considered one of the leading causes of liver disease nowadays.82 It comprises several liver disorders which impose health-care issues. Indeed, drug-induced hepatotoxicity may play an essential role in triggering acute liver failure.82 As the liver is a primary site for the detoxification reaction of most of the drug components, thus, it is a prime target for drug-induced damage.83 Drug-mediated liver damage and the associated inflammatory processes are generally characterized by the accumulation of inflammatory cells in the hepatic tissues as well as the cascade of activation of various inflammatory mediators leading to hepatic fibrosis.84 It is convinced that activation of quiescent Kupffer cells promotes the inflammatory processes which instigate hepatic damage.85 The acute inflammatory processes cause a substantial lethality as effective treatment option remains elusive. Existing reports have been made available to understand the mechanism of drug-induced hepatic inflammation, which demonstrates the critical factor for the development of drug-induced hepatic damage.86,87 Therefore, targeting the inflammation could solve the mystery of crosstalking mechanism between hepatic inflammation and damage progression. Various antioxidants are known to suppress inflammation and are expected to exhibit anti-inflammatory and hepatoprotective agents.88 Hence, the current study aimed to elucidate the anti-inflammatory and hepatoprotective action of an Indian variety of grape seed extract on drug-induced hepatic damage with particular emphasis on the regulation of Kupffer cell activation and associated signaling system.
Among the hepatotoxic drug, acetaminophen is widely studied. However, other pharmacological agents are also responsible for the development of hepatotoxicity.89–91 MTX, a folic acid analog, is used in treating arthritis with anti-proliferative, immunosuppressive, and anti-inflammatory activity.92 It is also commonly used to manage rheumatoid arthritis and psoriatic arthritis.92 The most common adverse effect of MTX usage includes hepatotoxicity,93 gastrointestinal toxicity,94 and nephrotoxicity.95 Numerous reports indicated that weekly usage of MTX enhanced the hepatic transaminase activity by 49%, suggesting the occurrence of hepatic anomalies.7 Hepatic inflammation and hepatic fibrosis were also noted in patients using MTX treatment.7 Due to its adverse effect, usage of MTX is suspended or discontinued in a patient having rheumatoid arthritis. Hence, the efficacy of this drug is limited to its adverse side effects. There is inadequate information regarding the mechanism of MTX-induced hepatotoxicity. Since the toxic effect of MTX has been reported to be mainly due to increased oxidative stress as a result of the production of free radical species,96 it is, therefore, hypothesized that the application of various antioxidants could reverse these anomalies. A growing body of evidence reported that fruit-based polyphenol97 and vitamins98 could suppress the MTX-induced damage followed by the progression of hepatic fibrosis. These compounds include crocin, berberine, and thiamine. Polyphenols and plant-derived vitamins are widely distributed in different types of botanicals, viz., fruits and vegetables. It forms an integral part of the diet and possesses a potent-free radical scavenging activity, limiting the activation of a free radical-mediated cellular signaling system.99–102 Studies have shown that pomegranate-fruit extract reversed the MTX-induced damage via redox-assisted signaling system activation modulation.63 Thus, the proposed research was to determine whether GSE could protect from MTX-induced liver damage and ascertain the molecular mechanism involved in the process.
Grape (Vitis vinifera) is considered one of the most widely cultivated fruits in the world.103 A growing body of evidence shows that the therapeutic activity of grapes is due to the presence of a plethora of bioactive components.104 The bioactive components of grape mainly exist in the seed and skin, despite the fact that the seed and skin are usually discarded in regular dietary intake and the grape juice industry.104 However, the use of grape seed as well as skin could be an excellent alternative as an exogenous antioxidant.105 The present study revealed the presence of putative fifteen bioactive components, which exhibited the basis of overall antioxidant potential and the hepatoprotective activity of GSE. GSE had a more significant amount of anthocyanidin than the other grape variety, and its glucoside component might be a good source of antioxidants.105 In the current study, the widely accepted mechanism behind hepatoprotection involved the amelioration of MTX-induced hepatic anomalies due to the free radical scavenging activity of GSE. This might relate to the high level of natural antioxidants in GSE. For example, GSE contained a significantly increased level of vitamin E (1.15–1.35 g/kg, above the Codex standard).106 Besides, GSE was found to have trilinolein (LLL, 43%), dilinoleoyl‐stearylglycerol (LSL, 19%) and dilinoleoyl‐palmitoylglycerol (LLP, 11%) as the predominant triacylglycerols,107 which are recognized natural antioxidants. GSE also contained many bioactive polyphenols (acylated anthocyanins and other flavonoids). Earlier reports demonstrated a crucial role of dietary trilinolein supplementation in the modulation of pro-inflammation.108,109 Primarily, it could inhibit the expression of pro-inflammatory markers. Our results indicate that the essential triacylglycerols present in GSE could serve as a potent hepatoprotector.
It has been reported earlier that the MTX generates oxidative stress in the liver.110 The functional aspects of the liver are reflected by the activity of its key enzymes, which are also considered “hepatic markers for disease indication”.111 The enhancement of liver toxicity markers, ALT, AST, and ALP upon MTX treatment, suggested the decreased functional ability of the liver. The increased liver weight and LDH activity confirmed the findings. The leakage of these cytosolic enzymes reflects hepatocyte dysregulation, which is associated with other consequences. Microscopic studies suggested an infiltration of inflammatory cells in degenerating hepatocytes and distorted hepatic architecture in MTX treatment which were in line with the biochemical findings. Several characteristic hepatic lessons, pyknosis, and RBC congestion were also evident in MTX treatment, suggesting the altered structural anomalies. Application of GSE in dual dosage (75 and 12 mg/kg) caused a significant reduction in liver toxicity indices and reversed the pathophysiological conditions consistent with the previous results.
Upon MTX treatment, the study demonstrated an augmentation of iROS generation in isolated hepatic cells, contributing to oxidative tissue damage as assessed by increased LPO.63 ROS is one of the critical players associated with the plethora of cellular anomalies.112 It is also regulating factor for the induction of the cellular signaling system and homeostasis. Cellular membranes, especially polyunsaturated fatty acids, are more susceptible to ROS, generating secondary lipid aldehydes that contribute to the LPO of various lipid membranes.113 The resultant damage of phospholipids in cell membranes can act as an adaptor molecule in triggering cell signaling systems associated with apoptosis and other inflammatory processes. The augmentation of ROS also suppressed the milieu of endogenous cellular antioxidants.114 Hence, it is of great interest to understand how MTX treatment produces ROS and how it is efficiently eliminated by GSE treatment to restore the antioxidant balance. As seen in the result, MTX-treatment enhanced the relative DCF fluorescence, an indication of ROS generation in hepatic cells as well as BODIPY fluorescence, suggesting the correlative activation of ROS-mediated LPO. On the other hand, concurrent activation of iROS and LPO also decreased the cellular endogenous antioxidant enzymes, SOD, and catalase, as evidenced by the lowered fluorescence activity of SOD-FITC and Catalase-FITC. Like ROS, RNS can be either detrimental or beneficial to the cellular system.115 Earlier, nitric oxide was identified as a signaling molecule contributing to blood vessel modulation. It is now considered a regulator of critical physiological processes connecting cellular toxicity with nitrosative stress.115 It can also mediate cellular damage by reacting with superoxide radical and peroxynitrite radical and activating an array of signaling pathways. MTX-induced cellular NO production was detected by DAF-FM fluorescence, which opened up the controversial role in inflammatory signaling system activation. The present study also demonstrated that the treatment with GSE in dual dosage (75 and 125 mg/kg) significantly alleviated the ROS and RNS generation and increased the expression of antioxidant enzymes, SOD, and catalase, ultimately reducing the LPO. The result was corroborated by the experimental observation of NAC treatment. This implied that GSE treatment might suppress the MTX-induced oxidative and nitrosative stress-assisted inflammatory development. Future works aim to address the latter and the underlying mechanism involved.
Kupffer cell is considered one of the vital components of the mononuclear phagocytic system and regulates hepatic and systemic responses to various pathogenic stimuli.116 It is regarded as a critical mediator of both liver injury and repair. Kupffer cells exhibit dual plasticity depending on the metabolic and immune environment. It can switch its phenotypic characteristics from the polarized pro-inflammatory M1 to the alternative M2 and vice versa upon the constant oxidative insults.116 A change in the functional activity of the Kupffer cell is associated with various disease states.116 A growing body of evidence demonstrated the heterogeneity of Kupffer cells. Mainly, livers are composed of two types of Kupffer cells, resident F4/80+CD68+ cells and recruited F4/80+CD11b+ cells.117 The recruited Kupffer cells from bone marrow to the liver exhibit significant pro-inflammatory activity upon various drug insults and trigger the development of inflammatory liver disease. Kupffer cells express multiple receptors for the complement system, including Complement receptors 1, 2, and 3 (CR1, CR2, and CR3). Clinically, the CR3 receptor has more significance in liver disease progression. CR3, also known as CD11b, is one of the primary surface markers in recruited Kupffer cells. Thus, drug-induced activation of CD11b+ Kupffer cells reflects the overall disease progression.118 Research demonstrated that exogenous molecule like the High Fat Diet increases CD11b+ Kupffer cells and inflammatory cytokine production in the mouse liver.119 Here, the study also depicted a similar incidence. MTX-treatment significantly increased the CD11b+ Kupffer cells, indicating the initiation of inflammatory development. The anti-inflammatory potential of GSE was preliminarily assessed as the augmentation of CD11b+ Kupffer cells reduced strikingly, suggesting the precise involvement of the anti-inflammatory mechanism. Next, it came to the question of how GSE reduced the CD11b+ Kupffer cell activation by an anti-inflammatory mechanism.
Almost several human diseases are strongly associated with inflammation, and the understanding of the progression of the disease reflects the precise mechanism of action of inflammation.120 A growing body of evidence suggests a crucial crosslink between inflammation and innate immune system dysregulation.120 Tissue injury can activate the cells of the innate immune system, which triggers inflammation. Surveillance mechanisms involve various systems. One of the essential mechanisms is the pattern recognition receptor (PRR) which leads to the activation of other downstream signaling systems.121 Most PRR responds to the various stimuli, especially pathogen-associated molecular pattern (PAMP) and host-derive danger-associated molecular pattern (DAMP), that triggers a plethora of redox-assisted transcription factors (NF-κB, CREB, IRF, AP1), leading to inflammation.121 The nucleotide-binding oligomerization (NOD)-like receptor (NLR) is a newly identified PRR that includes TLRs and C-type lectins.122 Both can generate severe immune responses upon binding with the selective ligands. NLR is one of the essential components of inflammasomes, a class of cytosolic protein complex that mediate the production of pro-inflammatory mediators. Four inflammasomes (NLRP1, Aim2, NLRP3, and NLRP4) are found in the cytosol containing the PRR that belongs to the NLR family.123 Among all types of inflammasomes, NLRP3 plays a vital role in regulating the inflammatory homeostasis in many common hepatic disorders and shaping immune reactions.122 NLRP3 is a multiprotein complex that consists of an NLRP3 scaffold, an adaptor protein, apoptosis speck-like protein (ASC), and the effector procaspase-1. The activation is initiated when the ASC associates with the NLRP3 scaffold upon the PRR activation and recruits procaspase-1 to generate active caspase-1. The active caspase-1 converts the immature pro-inflammatory cytokine precursor, pro-IL-1β, to biologically active IL-1β. Once activated, IL-1β can trigger a series of inflammatory responses by activating several cytokines and chemokines receptor.123 Recent research shows that NLRP3 activation in hepatocytes and parenchymal cells by several drugs molecule resulted in hepatocyte pyroptosis, fibrosis, and induction of pro-inflammatory signaling.124 The alteration in the hepatic milieu can exacerbate the various hepatic pathologies. In the present study, MTX significantly upregulated the NLRP3 along with the other two components, ASC and caspase 1, suggesting the activation of the inflammasome. Additionally, ROS has been suggested for inflammasome activation. It has been observed that ROS integrates different signals leading to the activation of inflammasomes. The augmented activation of pro-IL-1β was also observed in MTX-treatment, indicating the apparent connection between inflammasome activation and pro-inflammatory response. Moreover, MTX-induced ROS overproduction was associated with activation of NLRP3, ASC, and caspase-1, along with the increased release of IL-1β. These findings proved that activation of the ROS/NLRP3 inflammasome axis was essential in MTX-induced hepatic injury. On the other hand, it was evident that GSE in a dual dosage (75 and 125 mg/kg) suppressed the NLRP3 expression as well as ASC, caspase-1, and IL-1β expression, suggesting the anti-inflammatory effect possibly due to ROS elimination. The observation was also in line with the previous reports where grape seed proanthocyanidin (GSPE) alleviated NLRP3 activation in several model systems.125,126
Many experiments have indicated that TLR is a central mediator of the inflammatory response and establishes a crucial link between inflammation and fibrosis in chronic hepatic disorders.127 TLR has also been regarded as the direct activator of NF-κB in drug-induced anomalies.128 Recent reports demonstrated that the activation of the ROS/TLR4/MyD88/NF-κB axis plays a pivotal role in the regulation of inflammation as well as other pathological conditions.128 NF-κB, a redox-sensitive transcription factor, is involved in the regulation of transcription of a battery of pro-inflammatory genes, including cytokines and chemokines.89,129 Under the stressed condition, the regulator, nuclear factor of kappa light polypeptide gene enhancer in B-cells inhibitor, alpha (IκBα), is phosphorylated upon the concomitant activation of inhibitory kappa B kinases (IKK). The resultant action of IκBα phosphorylation release the masking effect on NF-κB and promotes its nuclear translocation subsequently to initiate the transcription of pro-inflammatory genes, IL-1β, interleukin-6 (IL-6), interleukin-8 (IL-8), and interleukin-18 (IL-18).129 It is also believed that the priming signal by TLR stimulation is also essential for transcriptional up-regulation of NLRP3.130 Here, the result depicted an upregulation of TLR4 and NF-κB found in MTX treatment. The activation of TLR4 and NF-κB was significantly inhibited by the application of GSE (75 and 125 mg/kg), indicating the probable anti-inflammatory mechanism through the suppression of the TLR4/NF-κB/NLRP3 axis.
The cytoprotective mechanism was also investigated in order to explore the underlying mechanism of the ameliorative effect of GSE on hepatic disorders. The Nrf2/antioxidant response element (ARE), a pivotal redox-regulated transcription factor signaling system, plays a vital role in cytoprotection by regulating the transcription of several genes related to antioxidant systems.131,132 HO-1, a phase-II detoxifying enzyme regulated by Nrf2, is the prime player in raising the endogenous antioxidant system.133 HO-1 catalyzes the reaction of heme to biliverdin, carbon oxide, and iron.131,134 Particularly, HO-1 has the most abundant ARE in promoting genes regulated by Nrf2 and has been regarded as essential in preventing hepatic disease caused by oxidative and nitrosative stress.133 Previously, MTX-induced down-regulation of Nrf2 was observed in the renal system.135 The current report demonstrated that Nrf2 expression was also reduced by MTX treatment in hepatic tissue, as observed in an immunofluorescence study. The total Nrf2 level was significantly lowered with respect to the control, whereas the application of a dual dosage of GSE (75 and 125 mg/kg) markedly augmented the expression. The observation was also compared with the sulforaphane-treated group. The diminished Nrf2 expression and translocation were confirmed by down-regulation of HO-1 and SOD levels that Nrf2 directly controls. Strikingly, HO-1 and SOD levels markedly enhanced upon GSE treatment (75 and 125 mg/kg), suggesting the precise mechanism of cytoprotection through the Nrf2/HO-1 pathway. From another point of view, a crosstalking mechanism exists between Nrf2 and NF-κB.136 Several phytochemicals are known to upregulate Nrf2 expression, which can terminate the NF-κB.137 On the other hand, in stress-mediated conditions, NF-κB can down-regulate Nrf2.138 It is reasonable to assume that upregulation of Nrf2 signaling following treatment with GSE suppressed ROS-provoked NF-κB/NLRP3 inflammasome activation.
The drug-induced liver injury leads to an alteration in the hepatic inflammatory response, which causes the activation of hepatic stellate cells that further secrete extracellular matrix protein-provoked hepatic fibrosis.139 The inflammatory response is the main driving force for fibrosis.17,140 A growing body of evidence suggests that concomitant activation of NLRP3 and NF-κB are critical for hepatic fibrosis.141 TGF-β, a key member of the TGF-β superfamily, plays a vital role in hepatic fibrosis progression.142 The elevated expression of TGF-β in affected organs is correlated with the matrix protein deposition. On the other hand, other fibrotic factors, including SMAD, fibronectin, and collagenase, are also responsible for the development of fibrosis.139 SMAD3, a key effector of the TGF-β signaling system, plays a pivotal role in pro-fibrosis and inflammation.143 Earlier studies demonstrated that suppression of SMAD3 inhibits type-I collagen expression and blocks epithelial myofibroblast transition. α-SMA is SMAD3 dependent, and SMAD3 directly binds to DNA sequences that regulate these target genes.139 Additionally, TGF-β triggers TIMP-1 by modulating SMAD3, thus inhibiting ECM degradation.139 Here, MTX-induced enhancement of hepatic pro-fibrotic factor was observed, indicating the involvement of pro-fibrogenic pathways. A significant augmentation of TGF-β and α-SMA expression and SMAD3, collagenase-I, and fibronectin level was also evident in MTX-treated hepatic cells, pointing out the same hypothesis. In contrast, GSE administration in a dual dose (75 and 125 mg/kg) markedly decreased the expression of TGF-β, α-SMA, SMAD3, fibronectin, and type-I collagenase, demonstrating the underlying mechanism of anti-fibrosis through the suppression of TGF-β/SMAD3 pathway.
Conclusion
This study has shown how GSE could ameliorate MTX-induced liver damage by modulating the redox-regulated signaling system and its ability to inhibit the NF-κB/NLRP3 inflammasome axis along with the activation of the Nrf2/HO-1 signaling system. It was demonstrated that GSE acted as a potent activator of Nrf2, and the consumption of GSE enhanced the liver antioxidant defense system (Figure 7). Furthermore, GSE reduced hepatic fibrosis through the down-regulation of the TGF-β/SMAD3 pathway. Based on the results, it may be concluded that GSE could be used as a prophylactic approach to diminish hepatic damage and for the treatment of oxidative stress-induced complications. Because of its significantly high levels of vitamin E and other bio-active phytochemicals, GSE could also be utilized by pharmaceutical or nutraceutical industries for the development of novel functional foods and supplements. This study has also opened up a new way for cost-effective treatment to manage liver cirrhosis.
![]() |
Figure 7 Schematic representation of possible mechanism of action of MTX-mediated hepatic anomalies and its prevention by GSE (75 and 125 mg/kg). |
Abbreviations
AICR TFase, 5-aminoimidazole-4-carboxamide ribonucleotide formyltransferase; ALP, Alkaline phosphatase; ALT, Alanine transaminases; AST, Aspartate transaminases; BODIPY, 5,5-Difluoro-5H-4λ5-dipyrrolo[1,2-c:2′,1′-f][1,3,2]diazaborinin-4-ylium-5-uide; BSA, Bovine serum albumin; CaCl2, Calcium chloride; COX-2, cyclooxygenase-2; DAF-FM DA, 4-amino-5-(methylamino)-2′,7′-difluorofluorescein diacetate; DAPI, 4′,6-diamidino-2-phenylindole; DCF, Dichlorofluorescein; DMEM, Dulbecco’s Modified Eagle Medium; FBS, Fetal bovine serum; FITC, Fluorescein isothiocyanates; GdCl3, gadolinium chloride; H2DCFDA, 2′,7′-dichlorofluorescein diacetate; HBSS, Hanks’ Balanced Salt Solution; HO-1, Heme oxygenase-1; IgG, Immunoglobulin G; IL-1β, Interleukin 1 beta; iNOS, inducible nitric oxide synthase; LC-MS, Liquid chromatography–mass spectrometry; LDH, Lactate dehydrogenase; MAPKs, Mitogen-activated protein kinases; NAC, N-Acetylcysteine; NF-κB, nuclear factor kappa-light-chain-enhancer of activated B cells; NLRP3, NLR family pyrin domain containing 3; NO, Nitric oxide; Nrf2, nuclear factor erythroid 2–related factor 2; PBS, Phosphate buffer saline; PE, Phycoerythrin; RNS, Reactive nitrogen species; SOD, Super oxide dismutase; TGF-β, Transforming growth factor-β; TLR4, Toll-like receptor 4; Tris-HCl, Tris(hydroxymethyl)aminomethane hydrochloride; UPLC-(ESI)-QToF-MS, Ultra performance liquid chromatography-Electro spray ionization-Quadrupole Time-of-Flight-Mass spectrometry; α-1AT, Alpha-1 antitrypsin; α-SMA, Alpha-Smooth Muscle Actin.
Ethics Approval and Informed Consent
All experimental protocols were performed as per the guidelines of the Institutional Animal Ethics Committee, CSIR-Indian Institute of Chemical Biology and approved by the Committee for the Purpose of Control and Supervision of Experiments on Animals (Ministry of Environment, Forests and Climate Change, The Government of India) (Approval No. IICB/AEC/Meeting/Feb/2018/1).
Acknowledgments
We are grateful to Debalina Chakraborty, Binayak Pal and Banasri Das of Central Instrumentation Facility, CSIR-Indian Institute of Chemical Biology for providing flow cytometer and confocal microscope facilities. Equally, we express our thankfulness to Suparna Banerjee for improving the language of the manuscript.
Author Contributions
All authors made a significant contribution to the work reported, whether that is in the conception, study design, execution, acquisition of data, analysis and interpretation, or in all these areas; took part in drafting, revising or critically reviewing the article; gave final approval of the version to be published; have agreed on the journal to which the article has been submitted; and agree to be accountable for all aspects of the work. Kaushik Banerjee and Krishna Das Saha are co-correspondence authors.
Funding
This work is supported by the National Medicinal Plant Board (Ministry of AYUSH, Government of India).
Disclosure
The authors declare no conflicts of interest in relation to this work.
References
1. Liang D, Guan Y, Zhu J, et al. Global research trends of drug-induced liver injury (Dili) in the past two decades: a bibliometric and visualized study. Ann Palliative Med. 2021;10(8):8651–8664. doi:10.21037/apm-21-981
2. Woods RL, Fox RM, Tattersall MH. Methotrexate treatment of advanced head and neck cancers: a dose response evaluation. Cancer Treat Rep. 1981;65(Suppl 1):155–159.
3. Ham JC, van Meerten E, Fiets WE, et al. Methotrexate plus or minus cetuximab as first-line treatment in a recurrent or metastatic (R/M) squamous cell carcinoma population of the head and neck (SCCHN), unfit for cisplatin combination treatment, a phase Ib-randomized Phase II study. Commence. 2022. doi:10.1002/hed.26053
4. O’Dell JR, Leff R, Paulsen G, et al. Treatment of rheumatoid arthritis with methotrexate and hydroxychloroquine, methotrexate and sulfasalazine, or a combination of the three medications: results of a two-year, randomized, double-blind, placebo-controlled trial. Arthritis Rheum. 2002;46(5):1164–1170. doi:10.1002/art.10228
5. Czarnecka-Operacz M, Sadowska-Przytocka A. The possibilities and principles of methotrexate treatment of psoriasis - The updated knowledge. Postepy Dermatol Alergol. 2014;31(6):392–400. doi:10.5114/pdia.2014.47121
6. Nesher G, Moore TL. Recommendations for drug therapy of rheumatoid arthritis in elderly patients. Clin Immunother. 1996;5(5):341–350. doi:10.1007/BF03259332
7. Conway R, Carey JJ. Risk of liver disease in methotrexate treated patients. World J Hepatol. 2017;9(26):1092–1100. doi:10.4254/wjh.v9.i26.1092
8. Abdelaziz AI, Mantawy EM, Gad AM, Fawzy HM, Azab SS. Activation of pCREB/Nrf-2 signaling mediates re-positioning of liraglutide as hepato-protective for methotrexate -induced liver injury (MILI). Food Chem Toxicol. 2019;132:110719. doi:10.1016/j.fct.2019.110719
9. Friedman B, Cronstein B. Methotrexate mechanism in treatment of rheumatoid arthritis. Joint Bone Spine. 2019;86(3):301–307. doi:10.1016/j.jbspin.2018.07.004
10. Herman S, Zurgil N, Deutsch M. Low dose methotrexate induces apoptosis with reactive oxygen species involvement in T lymphocytic cell lines to a greater extent than in monocytic lines. Inflamm Res. 2005;54(7):273–280. doi:10.1007/s00011-005-1355-8
11. Ochaion A, Bar-Yehuda S, Cohn S, et al. Methotrexate enhances the anti-inflammatory effect of CF101 via up-regulation of the A3adenosine receptor expression. Arthrit Res Ther. 2006;8(6):R169. doi:10.1186/ar2078
12. García DS, Saturansky EI, Poncino D, et al. Hepatic toxicity by methotrexate with weekly single doses associated with folic acid in rheumatoid and psoriatic arthritis. What is its real frequency? Ann Hepatol. 2019;2019:765–769. doi:10.1016/j.aohep.2019.01.011
13. Conway R, Carey J. Risk of liver disease in methotrexate treated patients. World J Hepatol. 2017;9:1092. doi:10.4254/wjh.v9.i26.1092
14. Kegel V, Pfeiffer E, Burkhardt B, et al. Subtoxic concentrations of hepatotoxic drugs lead to Kupffer cell activation in a human in vitro liver model: an approach to Study Dili. Mediators Inflamm. 2015;2015:640631. doi:10.1155/2015/640631
15. Heymann F, Hammerich L, Storch D, et al. Hepatic macrophage migration and differentiation critical for liver fibrosis is mediated by the chemokine receptor C-C motif chemokine receptor 8 in mice. Hepatology. 2012;55(3):898–909. doi:10.1002/hep.24764
16. Jacob A, Zhou M, Wu R, Halpern VJ, Ravikumar TS, Wang P. Pro-inflammatory cytokines from Kupffer cells downregulate hepatocyte expression of adrenomedullin binding protein-1. Biochim Biophys Acta. 2007;1772(7):766–772. doi:10.1016/j.bbadis.2007.03.010
17. Wynn TA. Cellular and molecular mechanisms of fibrosis. J Pathol. 2008;214(2):199–210. doi:10.1002/path.2277
18. Kolios G, Valatas V, Kouroumalis E. Role of Kupffer cells in the pathogenesis of liver disease. World J Gastroenterol. 2006;12(46):7413–7420. doi:10.3748/wjg.v12.i46.7413
19. Simón J, Casado-Andrés M, Goikoetxea-Usandizaga N, Serrano-Maciá M, Martínez-Chantar ML. Nutraceutical properties of polyphenols against liver diseases. Nutrients. 2020;12(11):3517. doi:10.3390/nu12113517
20. Michalska M, Gluba A, Mikhailidis DP, et al. The role of polyphenols in cardiovascular disease. Med Sci Monit. 2010;16(5):Ra110–9.
21. Di Meo F, Valentino A, Petillo O, Peluso G, Filosa S, Crispi S. Bioactive polyphenols and neuromodulation: molecular mechanisms in neurodegeneration. Int J Mol Sci. 2020;21(7):2564. doi:10.3390/ijms21072564
22. Liu K, Luo M, Wei S. The bioprotective effects of polyphenols on metabolic syndrome against oxidative stress: evidences and perspectives. Oxid Med Cell Longev. 2019;2019:6713194. doi:10.1155/2019/6713194
23. Cory H, Passarelli S, Szeto J, Tamez M, Mattei J. The role of polyphenols in human health and food systems: a mini-review. Front Nutr. 2018;5:87. doi:10.3389/fnut.2018.00087
24. Leitzmann C. Characteristics and health benefits of phytochemicals. Forsch Komplementarmed. 2016;23(2):69–74. doi:10.1159/000444063
25. Mu C, Sheng Y, Wang Q, Amin A, Li X, Xie Y. Potential compound from herbal food of Rhizoma Polygonati for treatment of COVID-19 analyzed by network pharmacology: viral and cancer signaling mechanisms. J Funct Foods. 2021;77:104149. doi:10.1016/j.jff.2020.104149
26. Ashktorab H, Soleimani A, Singh G, et al. Saffron: the golden spice with therapeutic properties on digestive diseases. Nutrients. 2019;11(5):943. doi:10.3390/nu11050943
27. Amin A, Farrukh A, Murali C, et al. Saffron and its major ingredients’ effect on colon cancer cells with mismatch repair deficiency and microsatellite instability. Molecules. 2021;26(13):3855. doi:10.3390/molecules26133855
28. Benassi E, Fan H, Sun Q, et al. Generation of particle assemblies mimicking enzymatic activity by processing of herbal food: the case of rhizoma polygonati and other natural ingredients in traditional Chinese medicine. Nanoscale Adv. 2021;3(8):2222–2235. doi:10.1039/D0NA00958J
29. Amin A, Bashir A, Zaki N, McCarthy D, Ahmed S, Lotfy M. Insights into glycan biosynthesis in chemically-induced hepatocellular carcinoma in rats: a glycomic analysis. World J Gastroenterol. 2015;21(20):6167–6179. doi:10.3748/wjg.v21.i20.6167
30. Amin A, Hamza A, Daoud S, et al. Saffron-based crocin prevents early lesions of liver cancer: in vivo, in vitro and network analyses. Recent Pat Anticancer Drug Discov. 2015;10. doi:10.2174/1574892810666151102110248
31. Al Hrout A, Baig B, Hilal-Alnaqbi A, Amin A. Cancer and biotechnology: a matchup that should never slowdown. In: Biotechnology and Production of Anti-Cancer Compounds. Springer; 2017:73–97.
32. Baig B, Halim SA, Farrukh A, Greish Y, Amin A. Current status of nanomaterial-based treatment for hepatocellular carcinoma. Biomed Pharmacother. 2019;116:108852. doi:10.1016/j.biopha.2019.108852
33. Al-Dabbagh B, Elhaty I, Murali C, Madhoon A, Amin A. Salvadora persica (Miswak): antioxidant and promising antiangiogenic insights. Am J Plant Sci. 2018;9:1228–1244. doi:10.4236/ajps.2018.96091
34. Kamal H, Jafar S, Mudgil P, Murali C, Amin A, Maqsood S. Inhibitory properties of camel whey protein hydrolysates toward liver cancer cells, dipeptidyl peptidase-IV, and inflammation. J Dairy Sci. 2018;101(10):8711–8720. doi:10.3168/jds.2018-14586
35. Pham DC, Shibu MA, Mahalakshmi B, Velmurugan BK. Effects of phytochemicals on cellular signaling: reviewing their recent usage approaches. Crit Rev Food Sci Nutr. 2020;60(20):3522–3546. doi:10.1080/10408398.2019.1699014
36. Chen H, Liu RH. Potential mechanisms of action of dietary phytochemicals for cancer prevention by targeting cellular signaling transduction pathways. J Agric Food Chem. 2018;66(13):3260–3276. doi:10.1021/acs.jafc.7b04975
37. Chun K-S, Raut PK, Kim D-H, Surh Y-J. Role of chemopreventive phytochemicals in NRF2-mediated redox homeostasis in humans. Free Radical Biol Med. 2021;172:699–715. doi:10.1016/j.freeradbiomed.2021.06.031
38. Yang J, Xiao YY. Grape phytochemicals and associated health benefits. Crit Rev Food Sci Nutr. 2013;53(11):1202–1225. doi:10.1080/10408398.2012.692408
39. Madrigal-Santillán E, Madrigal-Bujaidar E, Álvarez-González I, et al. Review of natural products with hepatoprotective effects. World J Gastroenterol. 2014;20(40):14787–14804. doi:10.3748/wjg.v20.i40.14787
40. Bagchi D, Garg A, Krohn RL, Bagchi M, Tran MX, Stohs SJ. Oxygen free radical scavenging abilities of vitamins C and E, and a grape seed proanthocyanidin extract in vitro. Res Commun Mol Pathol Pharmacol. 1997;95(2):179–189.
41. Terra X, Montagut G, Bustos M, et al. Grape-seed procyanidins prevent low-grade inflammation by modulating cytokine expression in rats fed a high-fat diet. J Nutr Biochem. 2009;20(3):210–218. doi:10.1016/j.jnutbio.2008.02.005
42. Sharma AK, Somkuwar RG, Bhange MA, Samarth RR. Evaluation of grape varieties for juice quality under tropical conditions of Pune region. Proc Natl Acad Sci. 2018;88(4):1517–1521. doi:10.1007/s40011-017-0894-4
43. Sharma A, Dagadkhair R, Somkuwar R. Evaluation of grape pomace and quality of enriched cookies after standardizing baking conditions. J AgriSearch. 2018;5:50–55. doi:10.21921/jas.v5i01.11134
44. Khoshbaten M, Aliasgarzadeh A, Masnadi K, et al. Grape seed extract to improve liver function in patients with nonalcoholic fatty liver change. Saudi J Gastroenterol. 2010;16(3):194–197. doi:10.4103/1319-3767.65197
45. Koley TK, Khan Z, Oulkar D, et al. High resolution LC-MS characterization of phenolic compounds and the evaluation of antioxidant properties of a tropical purple radish genotype. Arab J Chem. 2020;13(1):1355–1366. doi:10.1016/j.arabjc.2017.11.007
46. Koley TK, Khan Z, Oulkar D, et al. High resolution LC-MS characterization of phenolic compounds and the evaluation of antioxidant properties of a tropical purple radish genotype. Arab J Chem. 2017. doi:10.1016/j.arabjc.2017.11.007
47. Hassan SMA, Hassan SN, Maarof N. Clinical and Histopathological study of black and red grape seed extracts (Vitis Vinifera) effects on the Albino Mice. J Forensic Med. 2021;15:2024–2032.
48. Devi A, Jolitha AB, Ishii N. Grape seed proanthocyanidin extract (GSPE) and antioxidant defense in the brain of adult rats. Med Sci Monit. 2006;12(4):BR124–9.
49. Ommati MM, Amjadinia A, Mousavi K, Azarpira N, Jamshidzadeh A, Heidari R. N-acetyl cysteine treatment mitigates biomarkers of oxidative stress in different tissues of bile duct ligated rats. Stress. 2021;24(2):213–228. doi:10.1080/10253890.2020.1777970
50. Muriel P, Escobar Y. Kupffer cells are responsible for liver cirrhosis induced by carbon tetrachloride. J Appl Toxicol. 2003;23(2):103–108. doi:10.1002/jat.892
51. Marchetti C, Chojnacki J, Toldo S, et al. A novel pharmacologic inhibitor of the NLRP3 inflammasome limits myocardial injury after ischemia-reperfusion in the mouse. J Cardiovasc Pharmacol. 2014;63(4):316–322. doi:10.1097/FJC.0000000000000053
52. Sha T, Sunamoto M, Kitazaki T, Sato J, Ii M, Iizawa Y. Therapeutic effects of TAK-242, a novel selective Toll-like receptor 4 signal transduction inhibitor, in mouse endotoxin shock model. Eur J Pharmacol. 2007;571(2–3):231–239. doi:10.1016/j.ejphar.2007.06.027
53. Son M, Yoo J, Kwon C-I, et al. Associations of expressions of HBcAg and HBsAg with the histologic activity of liver disease and viral replication. Gut Liver. 2008;2:166–173. doi:10.5009/gnl.2008.2.3.166
54. Wu R, Zhou M, Wang P. Adrenomedullin and adrenomedullin binding protein-1 downregulate TNF-alpha in macrophage cell line and rat Kupffer cells. Regul Pept. 2003;112(1–3):19–26. doi:10.1016/S0167-0115(03)00018-1
55. Manna K, Khan A, Kr Das D, et al. Protective effect of coconut water concentrate and its active component shikimic acid against hydroperoxide mediated oxidative stress through suppression of NF-kappaB and activation of Nrf2 pathway. J Ethnopharmacol. 2014;155(1):132–146. doi:10.1016/j.jep.2014.04.046
56. Das K, Ghosh M. Structured DAG oil ameliorates renal injury in streptozotocin-induced diabetic rats through inhibition of NF-kappaB and activation of Nrf2 pathway. Food Chem Toxicol. 2017;100:225–238. doi:10.1016/j.fct.2016.12.033
57. Manna K, Khan A, Biswas S, et al. Naringin ameliorates radiation-induced hepatic damage through modulation of Nrf2 and NF-[small kappa]B pathways. RSC Adv. 2016;6(27):23058–23073. doi:10.1039/C6RA01102K
58. Kesh SB, Sikder K, Manna K, et al. Promising role of ferulic acid, atorvastatin and their combination in ameliorating high fat diet-induced stress in mice. Life Sci. 2013;92(17–19):938–949. doi:10.1016/j.lfs.2013.03.015
59. Hubbard GP, Stevens JM, Cicmil M, et al. Quercetin inhibits collagen-stimulated platelet activation through inhibition of multiple components of the glycoprotein VI signaling pathway. J Thrombosis Haemostasis. 2003;1(5):1079–1088. doi:10.1046/j.1538-7836.2003.00212.x
60. Han JH, Ju JH, Lee YS, et al. Astaxanthin alleviated ethanol-induced liver injury by inhibition of oxidative stress and inflammatory responses via blocking of STAT3 activity. Sci Rep. 2018;8(1):14090. doi:10.1038/s41598-018-32497-w
61. Yahfoufi N, Alsadi N, Jambi M, Matar C. The immunomodulatory and anti-inflammatory role of polyphenols. Nutrients. 2018;10(11):1618. doi:10.3390/nu10111618
62. Rider BJ. Methotrexate. In: Enna SJ, Bylund DB, editors. xPharm: The Comprehensive Pharmacology Reference. Elsevier; 2007:1–5.
63. Mukherjee S, Ghosh S, Choudhury S, et al. Pomegranate reverses methotrexate-induced oxidative stress and apoptosis in hepatocytes by modulating Nrf2-NF-kappaB pathways. J Nutr Biochem. 2013;24(12):2040–2050. doi:10.1016/j.jnutbio.2013.07.005
64. Poli G. Pathogenesis of liver fibrosis: role of oxidative stress. Mol Aspects Med. 2000;21(3):49–98. doi:10.1016/S0098-2997(00)00004-2
65. Allemann IB, Baumann LJ. Antioxidants used in skin care formulations. Skin Therapy Lett. 2008;13(7):5–9.
66. MacDonald GA, Bridle KR, Ward PJ, et al. Lipid peroxidation in hepatic steatosis in humans is associated with hepatic fibrosis and occurs predominately in acinar zone 3. J Gastroenterol Hepatol. 2001;16(6):599–606. doi:10.1046/j.1440-1746.2001.02445.x
67. Li S, Tan HY, Wang N, et al. The role of oxidative stress and antioxidants in liver diseases. Int J Mol Sci. 2015;16(11):26087–26124. doi:10.3390/ijms161125942
68. Wang H, Xue Y, Zhang H, et al. Dietary grape seed extract ameliorates symptoms of inflammatory bowel disease in IL10-deficient mice. Mol Nutr Food Res. 2013;57(12):2253–2257. doi:10.1002/mnfr.201300146
69. Stephens C, Andrade RJ, Lucena MI. Mechanisms of drug-induced liver injury. Curr Opin Allergy Clin Immunol. 2014;14(4):286–292. doi:10.1097/ACI.0000000000000070
70. Del Campo JA, Gallego P, Grande L. Role of inflammatory response in liver diseases: therapeutic strategies. World J Hepatol. 2018;10(1):1–7. doi:10.4254/wjh.v10.i1.1
71. Tacke F. Targeting hepatic macrophages to treat liver diseases. J Hepatol. 2017;66(6):1300–1312. doi:10.1016/j.jhep.2017.02.026
72. Lu YQ, Zhong S, Meng N, Fan YP, Tang WX. NLRP3 inflammasome activation results in liver inflammation and fibrosis in mice infected with Schistosoma japonicum in a Syk-dependent manner. Sci Rep. 2017;7(1):8120. doi:10.1038/s41598-017-08689-1
73. Kim HY, Kim SJ, Lee SM. Activation of NLRP3 and AIM2 inflammasomes in Kupffer cells in hepatic ischemia/reperfusion. FEBS J. 2015;282(2):259–270. doi:10.1111/febs.13123
74. Jo EK, Kim JK, Shin DM, Sasakawa C. Molecular mechanisms regulating NLRP3 inflammasome activation. Cell Mol Immunol. 2016;13(2):148–159. doi:10.1038/cmi.2015.95
75. Nakashima H, Ogawa Y, Shono S, et al. Activation of CD11b+ Kupffer cells/macrophages as a common cause for exacerbation of TNF/Fas-ligand-dependent hepatitis in hypercholesterolemic mice. PLoS One. 2013;8(1):e49339. doi:10.1371/journal.pone.0049339
76. Guo J, Friedman SL. Toll-like receptor 4 signaling in liver injury and hepatic fibrogenesis. Fibrogenesis Tissue Repair. 2010;3:21. doi:10.1186/1755-1536-3-21
77. Chen S, Zhu Y, Liu Z, et al. Grape seed proanthocyanidin extract ameliorates diabetic bladder dysfunction via the activation of the Nrf2 pathway. PLoS One. 2015;10(5):e0126457. doi:10.1371/journal.pone.0126457
78. Cuadrado A, Martín-Moldes Z, Ye J, Lastres-Becker I. Transcription factors NRF2 and NF-κB are coordinated effectors of the Rho family, GTP-binding protein RAC1 during inflammation. J Biol Chem. 2014;289(22):15244–15258. doi:10.1074/jbc.M113.540633
79. Amin A, Mahmoud-Ghoneim D. Texture analysis of liver fibrosis microscopic images: a study on the effect of biomarkers. Acta Biochim Biophys Sin. 2011;43(3):193–203. doi:10.1093/abbs/gmq129
80. Fabregat I, Moreno-Caceres J, Sanchez A, et al. TGF-beta signalling and liver disease. FEBS J. 2016;283(12):2219–2232. doi:10.1111/febs.13665
81. Carpino G, Morini S, Ginanni Corradini S, et al. Alpha-SMA expression in hepatic stellate cells and quantitative analysis of hepatic fibrosis in cirrhosis and in recurrent chronic hepatitis after liver transplantation. Dig Liver Dis. 2005;37(5):349–356. doi:10.1016/j.dld.2004.11.009
82. Katarey D, Verma S. Drug-induced liver injury. Clin Med. 2016;16(Suppl 6):s104–s109. doi:10.7861/clinmedicine.16-6-s104
83. Gu X, Manautou JE. Molecular mechanisms underlying chemical liver injury. Expert Rev Mol Med. 2012;14:e4. doi:10.1017/s1462399411002110
84. Kullak-Ublick GA, Andrade RJ, Merz M, et al. Drug-induced liver injury: recent advances in diagnosis and risk assessment. Gut. 2017;66(6):1154. doi:10.1136/gutjnl-2016-313369
85. Slevin E, Baiocchi L, Wu N, et al. Kupffer cells: inflammation pathways and cell-cell interactions in alcohol-associated liver disease. Am J Pathol. 2020;190(11):2185–2193. doi:10.1016/j.ajpath.2020.08.014
86. Woolbright BL, Jaeschke H. Mechanisms of inflammatory liver injury and drug-induced hepatotoxicity. Curr Pharmacol Rep. 2018;4(5):346–357. doi:10.1007/s40495-018-0147-0
87. Hamza AA, Lashin FM, Gamel M, Hassanin SO, Abdalla Y, Amin A. Hawthorn herbal preparation from Crataegus oxyacantha attenuates in vivo carbon tetrachloride -induced hepatic fibrosis via modulating oxidative stress and inflammation. Antioxidants. 2020;9(12):1173. doi:10.3390/antiox9121173
88. Zhu F, Du B, Xu B. Anti-inflammatory effects of phytochemicals from fruits, vegetables, and food legumes: a review. Crit Rev Food Sci Nutr. 2018;58(8):1260–1270. doi:10.1080/10408398.2016.1251390
89. Hamza AA, Heeba GH, Elwy HM, Murali C, El-Awady R, Amin A. Molecular characterization of the grape seeds extract’s effect against chemically induced liver cancer: in vivo and in vitro analyses. Sci Rep. 2018;8(1):1270. doi:10.1038/s41598-018-19492-x
90. David S, Hamilton JP. Drug-induced liver injury. US Gastroenterol Hepatol Rev. 2010;6:73–80.
91. El-Dakhly SM, Salama AAA, Hassanin SOM, Yassen NN, Hamza AA, Amin A. Aescin and diosmin each alone or in low dose- combination ameliorate liver damage induced by carbon tetrachloride in rats. BMC Res Notes. 2020;13(1):259. doi:10.1186/s13104-020-05094-2
92. Puig L. Methotrexate: new therapeutic approaches. Actas Dermosifiliogr. 2014;105(6):583–589. doi:10.1016/j.ad.2012.11.017
93. Vaidya B, Bhochhibhoya M, Nakarmi S. Efficacy of vitamin E in methotrexate-induced hepatotoxicity in rheumatoid arthritis: an open-label case-control study. Int J Rheumatol. 2020;2020:5723485. doi:10.1155/2020/5723485
94. Zhou B, Xia X, Wang P, et al. Induction and amelioration of methotrexate-induced gastrointestinal toxicity are related to immune response and gut microbiota. EBioMedicine. 2018;33:122–133. doi:10.1016/j.ebiom.2018.06.029
95. Kitamura M, Kitamura S, Fujioka M, et al. Methotrexate-induced acute kidney injury in patients with hematological malignancies: three case reports with literature review. Renal Replace Ther. 2018;4(1):39. doi:10.1186/s41100-018-0180-9
96. Mahmoud AM, Hussein OE, Abd El-Twab SM, Hozayen WG. Ferulic acid protects against methotrexate nephrotoxicity via activation of Nrf2/ARE/HO-1 signaling and PPARγ, and suppression of NF-κB/NLRP3 inflammasome axis. Food Funct. 2019;10(8):4593–4607. doi:10.1039/c9fo00114j
97. Li S, Tan HY, Wang N, Cheung F, Hong M, Feng Y. The potential and action mechanism of polyphenols in the treatment of liver diseases. Oxid Med Cell Longev. 2018;2018:8394818. doi:10.1155/2018/8394818
98. Licata A, Zerbo M, Como S, et al. The role of vitamin deficiency in liver disease: to supplement or not supplement? Nutrients. 2021;13(11):4014. doi:10.3390/nu13114014
99. Al Shamsi MS, Amin A, Adeghate E. Beneficial effect of vitamin E on the metabolic parameters of diabetic rats. Mol Cell Biochem. 2004;261(1–2):35–42. doi:10.1023/b:mcbi.0000028735.79172.9b
100. Al-Shamsi M, Amin A, Adeghate E. Effect of vitamin C on liver and kidney functions in normal and diabetic rats. Ann N Y Acad Sci. 2006;1084:371–390. doi:10.1196/annals.1372.031
101. Al-Shamsi M, Amin A, Adeghate E. Vitamin E decreases the hyperglucagonemia of diabetic rats. Ann N Y Acad Sci. 2006;1084:432–441. doi:10.1196/annals.1372.032
102. Hamza AA, Heeba GH, Hamza S, Abdalla A, Amin A. Standardized extract of ginger ameliorates liver cancer by reducing proliferation and inducing apoptosis through inhibition oxidative stress/ inflammation pathway. Biomed Pharmacother. 2021;134:111102. doi:10.1016/j.biopha.2020.111102
103. Lei Y, Chen Q, Chen J, Liu D. Potential ameliorative effects of grape seed-derived polyphenols against cadmium induced prostatic deficits. Biomed Pharmacother. 2017;91:707–713. doi:10.1016/j.biopha.2017.05.006
104. Sabra A, Netticadan T, Wijekoon C. Grape bioactive molecules, and the potential health benefits in reducing the risk of heart diseases. Food Chem X. 2021;12:100149. doi:10.1016/j.fochx.2021.100149
105. Gupta M, Dey S, Marbaniang D, Pal P, Ray S, Mazumder B. Grape seed extract: having a potential health benefits. J Food Sci Technol. 2020;57(4):1205–1215. doi:10.1007/s13197-019-04113-w
106. Songsermsakul P, Pornphairin E, Porasuphatana S. Comparison of antioxidant activity of grape seed extract and fruits containing high β-carotene, vitamin C, and E. Int J Food Properties. 2013;16(3):643–648. doi:10.1080/10942912.2011.561462
107. Ng T, Bekhit A, Fang EF, Wong J. Grape Seed (Vitis vinifera) Oils. Academic Press; 2016:455–462.
108. Luo T, Deng ZY, Li XP, Rao H, Fan YW. Triolein and trilinolein ameliorate oxidized low-density lipoprotein-induced oxidative stress in endothelial cells. Lipids. 2014;49(5):495–504. doi:10.1007/s11745-014-3889-4
109. Huang -S-S, Deng J-S, Lin J-G, Lee C-Y, Huang G-J. Anti-inflammatory effects of trilinolein from panax notoginseng through the suppression of NF-κB and MAPK expression and proinflammatory cytokine expression. Am J Chin Med. 2014;42(06):1485–1506. doi:10.1142/S0192415X14500931
110. Elango T, Dayalan H, Gnanaraj P, Malligarjunan H, Subramanian S. Impact of methotrexate on oxidative stress and apoptosis markers in psoriatic patients. Clin Exp Med. 2014;14(4):431–437. doi:10.1007/s10238-013-0252-7
111. Al-Shamsi M, Amin A, Adeghate E. Vitamin E ameliorates some biochemical parameters in normal and diabetic rats. Ann N Y Acad Sci. 2006;1084:411–431. doi:10.1196/annals.1372.033
112. Liu Z, Ren Z, Zhang J, et al. Role of ROS and Nutritional Antioxidants in Human Diseases. Front Physiol. 2018;9:477. doi:10.3389/fphys.2018.00477
113. Juan CA, Pérez de la Lastra JM, Plou FJ, Pérez-Lebeña E. The chemistry of Reactive Oxygen Species (ROS) revisited: outlining their role in biological macromolecules (DNA, lipids and proteins) and induced pathologies. Int J Mol Sci. 2021;22(9):4642. doi:10.3390/ijms22094642
114. Birben E, Sahiner UM, Sackesen C, Erzurum S, Kalayci O. Oxidative stress and antioxidant defense. World Allergy Organ J. 2012;5(1):9–19. doi:10.1097/WOX.0b013e3182439613
115. Weidinger A, Kozlov AV. Biological activities of reactive oxygen and nitrogen species: oxidative stress versus signal transduction. Biomolecules. 2015;5(2):472–484. doi:10.3390/biom5020472
116. Ju C, Tacke F. Hepatic macrophages in homeostasis and liver diseases: from pathogenesis to novel therapeutic strategies. Cell Mol Immunol. 2016;13(3):316–327. doi:10.1038/cmi.2015.104
117. Endo-Umeda K, Nakashima H, Komine-Aizawa S, Umeda N, Seki S, Makishima M. Liver X receptors regulate hepatic F4/80 (+) CD11b(+) Kupffer cells/macrophages and innate immune responses in mice. Sci Rep. 2018;8(1):9281. doi:10.1038/s41598-018-27615-7
118. Dixon LJ, Barnes M, Tang H, Pritchard MT, Nagy LE. Kupffer cells in the liver. Compr Physiol. 2013;3(2):785–797. doi:10.1002/cphy.c120026
119. Diehl KL, Vorac J, Hofmann K, et al. Kupffer cells sense free fatty acids and regulate hepatic lipid metabolism in high-fat diet and inflammation. Cells. 2020;9(10):2258. doi:10.3390/cells9102258
120. Hunter P. The inflammation theory of disease. The growing realization that chronic inflammation is crucial in many diseases opens new avenues for treatment. EMBO Rep. 2012;13(11):968–970. doi:10.1038/embor.2012.142
121. Takeuchi O, Akira S. Pattern recognition receptors and inflammation. Cell. 2010;140(6):805–820. doi:10.1016/j.cell.2010.01.022
122. Saxena M, Yeretssian G. NOD-like receptors: master regulators of inflammation and cancer. Review. Front Immunol. 2014;5. doi:10.3389/fimmu.2014.00327
123. Mangan MSJ, Olhava EJ, Roush WR, Seidel HM, Glick GD, Latz E. Targeting the NLRP3 inflammasome in inflammatory diseases. Nat Rev Drug Discov. 2018;17(8):588–606. doi:10.1038/nrd.2018.97
124. Wree A, Eguchi A, McGeough MD, et al. NLRP3 inflammasome activation results in hepatocyte pyroptosis, liver inflammation, and fibrosis in mice. Hepatology. 2014;59(3):898–910. doi:10.1002/hep.26592
125. Wan W, Zhu W, Wu Y, et al. Grape seed proanthocyanidin extract moderated retinal pigment epithelium cellular senescence through NAMPT/SIRT1/NLRP3 pathway. J Inflamm Res. 2021;14:3129–3143. doi:10.2147/JIR.S306456
126. Sheng K, Zhang G, Sun M, et al. Grape seed proanthocyanidin extract ameliorates dextran sulfate sodium-induced colitis through intestinal barrier improvement, oxidative stress reduction, and inflammatory cytokines and gut microbiota modulation. Food Funct. 2020;11(9):7817–7829. doi:10.1039/D0FO01418D
127. Yang L, Seki E. Toll-like receptors in liver fibrosis: cellular crosstalk and mechanisms. Review. Front Physiol. 2012;3. doi:10.3389/fphys.2012.00138
128. Li J, Jiang H, Wu P, et al. Toxicological effects of deltamethrin on quail cerebrum: weakened antioxidant defense and enhanced apoptosis. Environ Pollut. 2021;286:117319. doi:10.1016/j.envpol.2021.117319
129. Liu T, Zhang L, Joo D, Sun S-C. NF-κB signaling in inflammation. Signal Transduct Target Ther. 2017;2:17023. doi:10.1038/sigtrans.2017.23
130. Yang J, Wise L, Fukuchi K-I. TLR4 cross-talk with NLRP3 inflammasome and complement signaling pathways in Alzheimer’s disease. Review. Front Immunol. 2020;11. doi:10.3389/fimmu.2020.00724
131. Han B, Li S, Lv Y, et al. Dietary melatonin attenuates chromium-induced lung injury via activating the Sirt1/Pgc-1α/Nrf2 pathway. Food Funct. 2019;10(9):5555–5565. doi:10.1039/c9fo01152h
132. Lv Y, Jiang H, Li S, et al. Sulforaphane prevents chromium-induced lung injury in rats via activation of the Akt/GSK-3beta/Fyn pathway. Environ Pollut. 2020;259:113812. doi:10.1016/j.envpol.2019.113812
133. Loboda A, Damulewicz M, Pyza E, Jozkowicz A, Dulak J. Role of Nrf2/HO-1 system in development, oxidative stress response and diseases: an evolutionarily conserved mechanism. Cell Mol Life Sci. 2016;73(17):3221–3247. doi:10.1007/s00018-016-2223-0
134. Yang D, Yang Q, Fu N, et al. Hexavalent chromium induced heart dysfunction via Sesn2-mediated impairment of mitochondrial function and energy supply. Chemosphere. 2021;264(Pt2):128547. doi:10.1016/j.chemosphere.2020.128547
135. Mahmoud AM, Hozayen WG, Ramadan SM. Berberine ameliorates methotrexate-induced liver injury by activating Nrf2/HO-1 pathway and PPARγ, and suppressing oxidative stress and apoptosis in rats. Biomed Pharmacother. 2017;94:280–291. doi:10.1016/j.biopha.2017.07.101
136. Wardyn JD, Ponsford AH, Sanderson CM. Dissecting molecular cross-talk between Nrf2 and NF-κB response pathways. Biochem Soc Trans. 2015;43(4):621–626. doi:10.1042/BST20150014
137. Krajka-Kuźniak V, Baer-Dubowska W. Modulation of Nrf2 and NF-κB signaling pathways by naturally occurring compounds in relation to cancer prevention and therapy. Are combinations better than single compounds? Int J Mol Sci. 2021;22(15). doi:10.3390/ijms22158223
138. Bellezza I, Mierla AL, Minelli A. Nrf2 and NF-κB and their concerted modulation in cancer pathogenesis and progression. Cancers. 2010;2(2):483–497. doi:10.3390/cancers2020483
139. Xu F, Liu C, Zhou D, Zhang L. TGF-β/SMAD pathway and its regulation in hepatic fibrosis. J Histochem Cytochem. 2016;64(3):157–167. doi:10.1369/0022155415627681
140. Han B, Wang X, Wu P, et al. Pulmonary inflammatory and fibrogenic response induced by graphitized multi-walled carbon nanotube involved in cGAS-STING signaling pathway. J Hazard Mater. 2021;417:125984. doi:10.1016/j.jhazmat.2021.125984
141. Wu X, Dong L, Lin X, Li J. Relevance of the NLRP3 inflammasome in the pathogenesis of chronic liver disease. Review. Front Immunol. 2017;8. doi:10.3389/fimmu.2017.01728
142. Meng X-M, Nikolic-Paterson DJ, Lan HY. TGF-β: the master regulator of fibrosis. Nat Rev Nephrol. 2016;12(6):325–338. doi:10.1038/nrneph.2016.48
143. Li S, Zheng X, Zhang X, et al. Exploring the liver fibrosis induced by deltamethrin exposure in quails and elucidating the protective mechanism of resveratrol. Ecotoxicol Environ Saf. 2021;207:111501. doi:10.1016/j.ecoenv.2020.111501
© 2023 The Author(s). This work is published and licensed by Dove Medical Press Limited. The full terms of this license are available at https://www.dovepress.com/terms.php and incorporate the Creative Commons Attribution - Non Commercial (unported, v3.0) License.
By accessing the work you hereby accept the Terms. Non-commercial uses of the work are permitted without any further permission from Dove Medical Press Limited, provided the work is properly attributed. For permission for commercial use of this work, please see paragraphs 4.2 and 5 of our Terms.