Back to Journals » International Journal of Nanomedicine » Volume 18

Development of Curcumin and Piperine-Loaded Bio-Active Self-Nanoemulsifying Drugs and Investigation of Their Bioactivity in Zebrafish Embryos and Human Hematological Cancer Cell Lines
Authors Kazi M , Khan MF, Nasr FA , Ahmed MZ, Alqahtani AS, Ali MM, Aldughaim MS
Received 29 December 2022
Accepted for publication 25 March 2023
Published 6 April 2023 Volume 2023:18 Pages 1793—1808
DOI https://doi.org/10.2147/IJN.S400330
Checked for plagiarism Yes
Review by Single anonymous peer review
Peer reviewer comments 3
Editor who approved publication: Prof. Dr. Anderson Oliveira Lobo
Supplementary video 1 - Curr-circulation [ID 400330].
Views: 71
Mohsin Kazi,1 Muhammad Farooq Khan,2 Fahd A Nasr,3 Mohammad Z Ahmed,3 Ali S Alqahtani,3 Meser M Ali,4 Mohammed S Aldughaim5
1Department of Pharmaceutics, College of Pharmacy, King Saud University, Riyadh, 11451, Kingdom of Saudi Arabia; 2Department of Zoology, College of Science, King Saud University, Riyadh, 11451, Kingdom of Saudi Arabia; 3Department of Pharmacognosy, College of Pharmacy, King Saud University, Riyadh, 11451, Kingdom of Saudi Arabia; 4Department of Neurosurgery, Henry Ford Health, Detroit, MI, 48202, USA; 5Research Center, King Fahad Medical City, Riyadh Second Health Cluster, Riyadh, 11525, Kingdom of Saudi Arabia
Correspondence: Mohsin Kazi; Mohammed S Aldughaim, Email [email protected]; [email protected]
Purpose: Curcumin (CUR) and piperine (PP) are bioactive compounds with prominent pharmacological activities that have been investigated for the treatment of various diseases. The aim of the present study is to develop Bio-SNEDDS for CUR and PP as a combined delivery system for cancer therapy.
Methods: CUR and PP loaded Bio-SNEDDSs with varying compositions of bioactive lipid oils, surfactants, and cosolvents were prepared at room temperature. Bio-SNEDDSs were characterized using a Zetasizer Nano particle size analyzer and further examined by transmission electron microscopy (TEM) for morphology. The in vivo toxicity of the preparations of Bio-SNEDDS was investigated in wild-type zebrafish embryos and cytotoxicity in THP-1 (human leukemia monocytic cells), Jurkat (human T lymphocyte cells) and HUVEC (non-cancerous normal) cells.
Results: Bio-SNEDDSs were successfully developed with black seed oil, Imwitor 988, Transcutol P and Cremophor RH40 at a ratio of 20/20/10/50 (%w/w). The droplet size, polydispersity index and zeta potential of the optimized Bio-SNEDDS were found to be 42.13 nm, 0.59, and − 19.30 mV, respectively. Bio-SNEDDS showed a spherical structure evident by TEM analysis. The results showed that Bio-SNEDDS did not induce toxicity in zebrafish embryos at concentrations between 0.40 and 30.00 μg/mL. In TG (fli1: EGFP) embryos treated with Bio-SNEDDS, there was no change in the blood vessel structure. The O-dianisidine staining of Bio-SNEDDS treated embryos at 48 h post-fertilization also showed a significant reduction in the number of blood cells compared to mock (DMSO 0.1% V/V) treated embryos. Bio-SNEDDS induced significant levels of cytotoxicity in the hematological cell lines THP-1 and Jurkat, while low toxicity in normal HUVEC cell lines was observed with IC50 values of 18.63± 0.23 μg/mL, 26.03 ± 1.5 μg/mL and 17.52 ± 0.22 μg/mL, respectively.
Conclusion: Bio-SNEDDS exhibited enhanced anticancer activity and could thus be an important new pharmaceutical formulation to treat leukemia.
Graphical Abstract:
Keywords: curcumin, piperine, bioactive self-nanoemulsifying drug delivery systems, leukemia, combination therapy
Graphical Abstract:
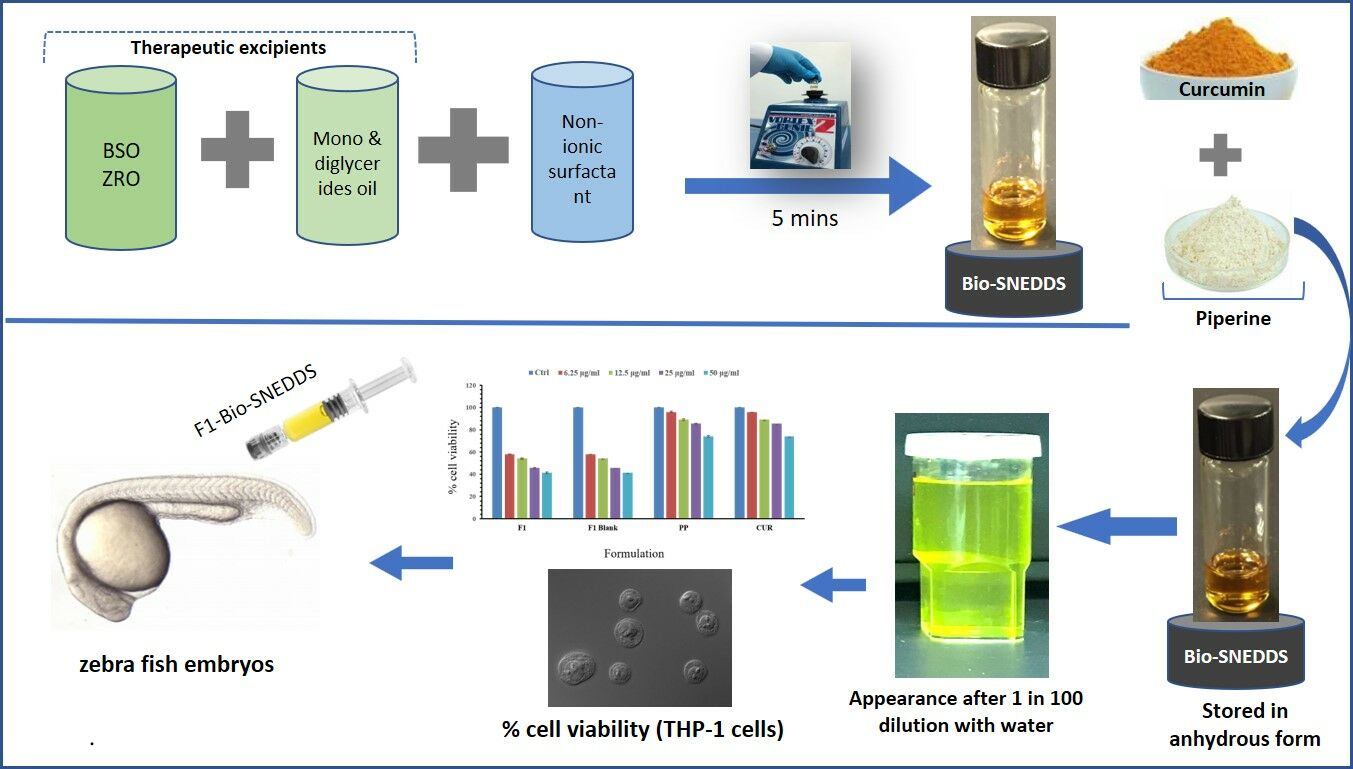
Introduction
Curcumin (CUR) is a bioactive food compound that has attracted considerable interest for its beneficial potential for human health. This includes anti-aging, anti-tumor, anti-inflammatory, anti-mutagenic, anti-oxidative and radio-sensitizing properties.1 CUR is a naturally occurring polyphenolic compound found in the turmeric plant (Curcuma longa L.). Piperine (PP) is a pungent alkaloid found in black pepper and it exhibits several beneficial biological effects such as antimicrobial action, as well as physiological effects that can contribute to general human health, including immunomodulatory, hepatoprotective, antioxidant, antimetastatic, and antitumor effects.2 Evidence from clinical studies has demonstrated the remarkable antioxidant, antitumor, immunomodulatory and drug availability-enhancing characteristics of both of these compounds.3 Because most of the CUR-based nanoformulation evidence is still in the conceptual stage, there are still numerous issues or impediments to suggesting nanocurcumin as a possible therapeutic option. However, a scientific gap exists due to a paucity of data from CUR-based nanoformulations; hence, future dosage formation techniques are necessary to promote CUR as a viable anti-cancer alternative. Based on these characteristics and the therapeutic potential of CUR and PP, they have been investigated for incorporation into drug formulations to enhance drug response, as well as into those that could be used as adjunct therapy to enhance the bioavailability of various (chemo)therapeutic drugs.4
Recently, the combination of therapeutic agents with natural compounds as excipients has gained great interest for enhancing their efficacy.5 Most importantly, PP has been reported to possess effective chemo protective activity that could act via several mechanisms such as enhancing antioxidant activities, increasing the level and activity of detoxifying enzymes and suppressing stem cell self-renewal.6,7 Moreover, PP has been found to inhibit the proliferation and survival of various cancer cell lines via modulating cell cycle progression and exhibiting anti-apoptotic activity, respectively.8,9 PP has exhibited anti-MDR activities and is able to modulate the MDR phenotype in some experimental models, such as cancers of the breast, lung, colon and lymphoma.7
Several studies have reported selective cytotoxic activity of PP on cancer cells compared with normal cells.9,10 Most of the studies suggested PP as a promising candidate for further development, particularly in clinical trials. These studies provide a scientific rationale for the co-administration of PP with CUR to investigate their therapeutic efficacy against several cancer cell lines.10
Previous studies reported that PP enhances the extent of absorption,6 serum concentration, and bioavailability of CUR in rats as well as humans.11 In particular, concomitant administration of PP along with CUR increases the bioavailability of CUR by 2000% compared to CUR alone in humans.12 In addition, the combination of PP with CUR showed significant potentiation of its neurotransmitter enhancing (serotonin and dopamine) effects, anti-immobility, and inhibitory effects on monoamine oxidase compared to the effects of CUR alone.13
In this investigation, an interesting approach taken for improving the efficacy and delivery of CUR (Figure 1A) was co-administration with PP (Figure 1B) using thymoquinone as the carrier excipient (Figure 1C). Thymoquinone is a phytochemical compound found in black seed oil. We have developed a novel self-nanoemulsifying drug delivery system (SNEDDS) containing black seed oil to serve as a non-toxic tool to enhance and control the release of CUR and PP.
![]() |
Figure 1 Chemical structures of (A) curcumin, (B) piperine and (C) thymoquinone. |
Bio-SNEDDSs have been characterized in vitro and preclinically applied in zebrafish embryos. Zebrafish embryos are used because they are a powerful animal model of haematopoiesis. Zebrafish embryos are transparent, which allows direct visualization of hematopoietic cells in live animals. Zebrafish embryos are permeable to compounds, which have been dissolved in water and due to their small size, they can be housed in 96-well plates, and thus can be easily used for high-throughput chemical screening. A whole-animal model chemical screening approach significantly reduces encountering common issues such as drug toxicity and off-target side effects that arise during late stages of drug development in cell-based drug screening. Thus, zebrafish embryos provide an excellent whole-animal screening model and are ideal for conducting the initial stages of drug screening, which could lessen research expenses as well as extensive labour and timings that are necessary when using other models. Human blood diseases have been modelled in zebrafish, and small-molecule screens in such models have the potential to reveal new drugs for human disease treatment.14 The zebrafish has another unique advantage regarding haematopoiesis that no other vertebrate has, in that zebrafish embryos can survive without active circulation and get oxygen from the water by passive diffusion, for the first week. However, lack of haematopoiesis results in lethality in mouse embryos, thus zebrafish provides an excellent experimental setup to identify compounds that target haematopoiesis.
Leukemia is considered one of the most lethal diseases and extremely aggressive malignancies with a high recurrence and mortality rate worldwide. It is a leading cause of death and traditional treatments induce significant medical toxic effects and unpleasant adverse reactions. Chemotherapies and other targeted therapies show insignificant clinical outcomes with little effect on the overall patient survival rate. Conventional treatment of leukemia is often hindered due to the adverse effects of available chemotherapy. Therefore, effective medications that are extremely specific with few adverse consequences are urgently needed. Combination therapy using black seed oil, Cur and PP can produce greater therapeutic effects and reduce toxicity.15,16 Co-administration of black seeds, and turmeric showed enhanced efficacy in preventing inflammation, cancer and metabolic syndrome (MS) in fructose-fed rats.17 Bio-SNEDDSs represent an orally ingested safe formulation as isotropic solutions of oil, surfactant, cosurfactant, and the drug in a solubilized state. The spontaneous nanoemulsions formed by Bio-SNEDDS are less than 200 nm in droplet size, which provides a large interfacial surface area for drug absorption. Apart from solubilization, oral bioavailability augmentation is also achieved by inducing intestinal lymphatic transport and hence, reducing first-pass metabolism, enhancing intestinal permeability and reducing metabolism and P-glycoprotein-mediated efflux.18
Black seed oil (BSO) has been widely used in traditional Arabic medicine for the treatment of arthritis, lung diseases and hypercholesterolemia.19 Some of the reported pharmacological properties of BSO include hypotensive, anti-nociceptive, uricosuric, choleretic, anti-fertility, anti-diabetic, anti-histaminic, anti-oxidant, anti-inflammatory, anti-microbial, anti-tumor and immunomodulatory effects.20 Hence, this study demonstrates the therapeutic superiority of the combination of CUR and Thymoquinone (TQ) at low doses over individually used doses, in improving leukemia treatment features.21
Materials and Methods
Curcumin (CUR, purity = 99.5%) was supplied by Enzo Life Sciences (Lausen, Switzerland). Piperine (PP, purity = 99.8%) was obtained from Sigma Aldrich (MO, USA). Imwitor 988 (I988, medium-chain mono and diglycerides) and non-ionic surfactant Tween 85 (T85) were obtained from Sasol, GmbH (Werk Witten, Witten, Germany). Cremophor RH40 (Polyoxyl 40 Hydrogenated Castor Oil, CrRH40) was supplied by BASF (Ludwigshafen, Germany). Transcutol P (TcP) was generously supplied by Gattefossé (Saint priest, France). BSO was obtained from the seeds of Nigella sativa (N. sativa) Linn. (black seeds), family Ranunculaceae and Zanthoxylum rhetsa seed oil (ZRO) obtained from dried fruits of Roxb DC plant that belongs to the family Rutaceae by the cold press/steam distillation method. Both BSO and ZRO were collected from Kaligonj, Gazipur, Bangladesh. Highly purified Milli-Q water was used from a Milli-Q Integral Water Purification System (Millipore, Bedford, MA). All other chemicals and reagents used in the studies were analytically pure.
Reagents for Cell Culture and the Source Cells (HUVEC, THP1, and Jurkat Clone E6-1 Cells)
The RPMI-1640 media, penicillin, streptomycin, dimethyl sulfoxide (DMSO), and trypan blue (TB) were purchased from Sigma Aldrich (Mo, USA). Fetal calf serum (FCS) was purchased from Gibco (USA). MTT (3-[4, 5-dimethylthiazol-2, 5-diphenyltetrazolium bromide]) reagent was purchased from Invitrogen (USA). Cell culture microtiter plates, and tubes (Corning Falcon, USA). Human umbilical vein endothelial cells (HUVEC), Jurkat clone E6-1 cells and THP-1 human monocytic cells were purchased from American Type Culture Collection (16549; Cat no. PCS-100-010; ATTCC, Manassas, VA, USA) and (ATCC-TIB202), respectively.
Animal
Adult zebrafish were raised and maintained in the animal facility of Bioproducts research chair, College of Science, Department of Zoology, following the guidelines described in the zebrafish book.22
Plant Material and Extraction of Bioactive Oils
The methods of collection, extraction, and standardization of BSO and ZRO were explained in detail in our previous publication.12 Both BSO and ZRO are mainly long-chain triglycerides/fatty acids containing several active phytochemicals. The extracted oil was filtered and stored in a screw-capped amber glass bottle for further use in SNEDDS development.
Bio-SNEDDS Development and Optimisation
The excipients used to develop Bio-SNEDDS in this research were plant-derived oils, hydrophobic/hydrophilic surfactants and water-soluble cosolvents. The different ratios of oils and surfactants were blended to represent two formulation systems “SNEDDS-1” (F1) and “SNEDDS-2” (F2). Both Bio-SNEDDSs were formulated using just six components: oils-BSO, ZRO, I988, surfactants-T85, CrRH40, and cosolvent-TcP, and by changing their combinations. Based on our previous studies, BSO and ZRO were used as plant derived bioactive oils for adding the synergistic effects. In brief, F1-Bio-SNEDDS was prepared using 20% BSO and 20% I988, as oil phase along with 10% water-soluble cosolvent TcP. Water-soluble surfactant CrRH40 (50% w/w) was added to the oil phase by a vortex mixture to ensure it is homogenized. F1-Bio-SNEDDS contained mostly water-soluble components. On the other hand, F2 Bio-SNEDDS was prepared with 35% ZRO and 15% I988 as oil phase. The oil phase was mixed with 50% water-insoluble surfactant T85. Oils and surfactant were blended without any water-soluble cosolvent in this formulation. F2-Bio-SNEDDS is developed with water-insoluble lipid materials. The representative formulations were developed in liquid form and incorporated with CUR and PP for maximum solubility. The resulting Bio-SNEDDSs were kept in a screw capped glass vial until further experimental use.
Viscosity Measurement of the SNEDDS
The viscosity of Bio-SNEDDSs was monitored by standard rheological techniques. The anhydrous formulation was diluted with Milli-Q water at a 1:1000 dilution factor. The diluted sample solution was filled in a 50mL chamber and then measured using a small sample adapter of a Brookfield cone and plate rheometer (Model LV2, Brookfield Engineering Laboratories, Stoughton, MA, USA) at room temperature (25°C). The sample was repeated in triplicate.
Droplet Size, Polydispersity Index (PDI) and Zeta Potential of Bio-SNEDDS
The droplet size distribution and PDI of the optimized Bio-SNEDDS were measured utilizing a particle size analyzer (Zetasizer Nano, Model ZEN3600, Malvern, Yorkshire, UK). In addition, the surface charge of the dispersing Bio-SENDDS formulation was determined by Zeta Potential measurement. The samples for both droplet size and zeta potential were prepared by diluting anhydrous formulation with water at a ratio of 1:1000 v/v and mixed for 1 min before being transferred into cuvettes for testing. The experiments were performed in triplicate.
Transmission Electron Microscopy (TEM) Analysis
TEM (JEOL JEM1010, JEOL Ltd., Akishima, Tokyo, Japan) was used for analyzing the morphology of CUR- and PP-loaded Bio-SNEDDSs. A drop of diluted sample (with water) was placed on a 300 mesh carbon coated copper grid. Excess liquid was removed using filter paper, and the grid was left to air dry. Then, a drop of 1% phosphotungstic acid in water was added to the grid, left for 5 min to settle down and dried as previously noted. Finally, the dried grid was visualized at an operating voltage of 80 kV, and images of the Bio-SNEDDS were captured.3
CUR and PP Loading into Bio-SNEDDS
CUR and PP loading was performed according to the maximum equilibrium solubility estimated from our previous studies.12 CUR and PP were loaded at 60% equilibrium solubility to make sure that the Bio-SNEDDS remained stable (no drug precipitation/degradation occurred) upon aqueous dispersion. Bio-SNEDDSs (both F1 and F2) were loaded with CUR and PP as combined dosage form throughout the current experimental studies.
Cell Proliferation (MTT) Assay
Cell Cultures
THP-1 (human leukemic cells) and Jurkat clone E6-1 cells were cultured at 37°C in 5% CO2 in RPMI-1640 media supplemented with 10% FCS, penicillin (100 IU/mL), and streptomycin (100 g/mL). The cells were seeded at a density of 2×104 cells per well and then incubated with various doses (0, 6.25, 12.5, 25 and 50 µg/mL) of drug formulation for 24 h, while untreated cells served as control. All the experiments were carried out in triplicate. HUVEC (normal) cells were cultivated in DMEM with 10% FBS and 1% penicillin –streptomycin antibiotic solution. The antineoplastic activity of CUR, PP, F1 (SNEDDS-1), F2 (SNEDDS-2) and C1-C2 (control-combination of pure CUR and PP) was measured in vitro by MTT assay according exactly as reported previously Nasr FA et al.23 Briefly, cells were cultured in the presence of different formulations of the SNEDDS at various concentrations (0–100 µg/mL) for 48 h. After incubation, 10 µLof 5 mg/mL MTT solution (prepared in PBS) was added to each well and incubated for an additional 4 h. Finally, the reduced MTT (formazan product) was solubilized by acidified isopropanol (0.01N HCl). The absorbance was measured at 540 nm; wells with untreated cells were utilized as controls and doxorubicin was used as a positive control. The IC50 values were calculated from the dose–response curve using OriginPro 8.5 software.
Cell viability (%) = Mean OD/Control OD x 100
In vivo Assay Using Zebrafish Embryo
We screened the compounds in zebrafish embryos to determine the biological activity and safety of the compounds in a whole animal.
Animal Treatment
The wild type (AB Tubingen) and transgenic Tg (Fli1: EGFP) zebrafish embryos were obtained by natural pairwise mating. The fertilized embryos were syphoned using sterile plastic pasteur pipettes and dead and unfertilized embryos were removed. Adult zebrafish were maintained by following local and international guidelines for the use and care of laboratory animals. This study used zebrafish larvae less than 5 days post fertilization (dpf). It has been internationally accepted that zebrafish larvae until the age of 6 days post fertilization (pdf) are not considered fully grown animals and are exempt from ethical approval from institutional review boards (IRBs), as declared in Lackmann et al, 2018a, and Strahle et al, 2012.24,25
Preparation of Compounds to Treat Zebrafish Embryos
A stock solution of 25 mM was made by dissolving the F1-SNEDDS, pure CUR and pure PP in molecular biology grade DMSO. Synchronous stage embryos were sorted, and embryos at the shield stage were transferred to 6-well cell culture plates (three wells for each treatment) and exposed to serial dilutions (0.1,0.5,1.00, 5, 15, and 45 µM) of each compound in a total volume of 2mL of embryo medium. The response of the embryos toward mortality and embryonic toxicity (teratogenicity) was monitored after 12 h. Most of the embryos died at higher concentrations, while the live embryos remained exposed to the compound for 3 days, but the embryo medium was replaced with fresh medium every day. Embryonic mortality and development were monitored every 24 h until the end of the experiment. The experiment was repeated at least three times (triplicate biological repeats) by using a new batch of embryos every time. The LC50 for zebrafish embryonic toxicity was calculated using an updated Probit analysis using the Finney method.26
Haemoglobin Staining of Live Zebrafish Embryos
To determine the effect of the F1-SNEDDS formulation on blood formation, O-dianisidine was used to stain red blood cells. For this purpose, embryos at the shield stage were treated with sublethal concentration of F1 (0.4µg/mL). The embryos were grown up to 20 somite stage and then a Phenylthiourea (Sigma Aldrich) final concentration 0.003% was added to the embryo medium to stop the pigmentation in developing embryos. Embryos were stained in the dark for 30 min at room temperature in a solution containing o-dianisidine (0.6 mg/mL), 0.01 M sodium acetate (pH 4.5), 0.65% H2O2, and 40% (vol/vol) ethanol. Once stained, embryos were washed with RO water and then fixed in 4% paraformaldehyde for at least 1 h at room temperature. Embryos were washed with phosphate-buffered saline (PBS) containing 0.1% Tween-20 and then fixed in 4% paraformaldehyde for at least 3 h at 4°C.
Statistical Analysis
The data were analysed as ANOVA (One-way analysis of variance by IBM SPSS statistics 26®) followed by Post Hoc Tests (LSD) and applied to compare the IC50 of pure drugs and Bio-SNEDDS formulations. P values <0.05 were considered significant throughout the study.
Results and Discussion
Bio-SNEDDS Formulation Design and Optimisation
The lipid excipients used to formulate the Bio-SNEDDS and their combinations in the F1 and F2 systems are shown in Table 1. In the current studies, the formulations were prepared following a lipid formulation classification system (LFCS).27 LFCS framework was first introduced by Colin Pouton to develop SNEDDS formulation with a minimum number of excipients; thus, avoiding trial and errors. Usually, LFCS Type III and IV systems produce SNEDDS formulation with more than 50% surfactants. F1 and F2-Bio-SNEDDSs in this work belong to Type III–IV classification systems.
![]() |
Table 1 Composition of the “F1” and “F2” Bio-SNEDDS Formulations Developed in the Studies |
The F1 formulation system contained 40% oil [20% BSO, 20% I988], 10% cosolvent [TcP] and 50% water-soluble surfactant (CrRH40, HLB 14–16), whereas the F2 formulation system consisted of 50% oil [35% ZRO, 15% I988] and 50% water-insoluble surfactant [T85, HLB 11], respectively. The F1 system has 10% less oil quantity than F2 but is compensated with a 10% water-soluble cosolvent. In addition, the F1 system is composed of the water-soluble surfactant Cremophor RH40. In comparison, the F1 system was composed of more polar (10% transcutol P- water-soluble cosolvent) excipients than the F2 system.
The formulations that are transparent, homogeneous and take less time to disperse (ie, less than 1 min), are more likely to be efficient and hence suitable to be formulated as Bio-SNEDDS in the current study. In addition, to meet the SNEDDS criteria, the droplet size of the dispersed Bio-SNEDDS should be less than 200nm. In the current study, both the F1 and F2 formulations were qualified as Bio-SNEDDS; however, F1 has a more transparent appearance when loaded with CUR and PP (Figure 2-inset). No signs of coalescence or phase separation were noticed in their preparations.
The reduction in the free energy required for emulsion formation consequently improves the stability of the self-nanoemulsifying formulation.28 Therefore, the selection of oil and surfactant/cosolvent (focused on safety), and the mixing ratio of oil to surfactant/cosolvent (focused on stability), play an important role in the formation of Bio-SNEDDSs and their fate upon aqueous dispersion in the intestine. FSO and ZRO are known for their safety for oral administration. Using surfactants with HLB (hydrophilic lipophilic balance) values close to those needed for oils results in the formation of more stable emulsions. The current studies used non-ionic surfactants of Tween 85 (HLB 11) and Cremophor RH40 (HLB 16), respectively. Both the F1 and F2 Bio-SNEDDSs are considered safe due to the nonirritant and nontoxic ingredients used in systems.
Appearance of SNEDDS Upon Aqueous Dispersion and Viscosity Measurement
SNEDDS formulation is needed to visually assess the self-emulsification properties of the formulation.29,30 A maximum dilution (1 in 100 dilution) factor was used for the SNEDDS dosage form to mimic the intestinal dilution conditions. Visual assessment helped to understand which formulation produced nanodroplets upon dilution with intestinal contents. The F2 formulation contained mostly water-insoluble excipients, thus forming a self-emulsified dispersion in aqueous media. Typically, this formulation produced turbid emulsions spontaneously when diluted. When the water-soluble surfactant CrRH40 and cosolvent TcP were used in F1 instead of T85, the resultant dispersion was transparent. It is likely that the formulation containing water-soluble surfactants produced a fine emulsion, which generally formed a transparent solution of micelles on aqueous dispersion.
The viscosity of anhydrous Bio-SNEDDSs were monitored by standard rheological techniques to confirm that they met the requirements of SNEDDS characteristics. The viscosity of formulations is dependent on the use of lipid oils and surfactants in the delivery systems. The optimized formulation of drug-free F1 and drug-loaded F1 has a minimum viscosity of 0.68 Cp and 0.71 Cp, respectively. On the other hand, F2 drug-free and drug-loaded SNEDDSs have higher viscosity compared to the F1 system. The results of viscosity are shown in Figure 2.
Droplet Size, PDI and Zeta Potential of Bio-SNEDDS
The droplet size analysis of the F1 and F2 SNEDDS formulations showed low droplet sizes upon dilution with water. As reported earlier, self-emulsifying efficiency and the rate and extent of drug release in the intestine are strongly associated with the mean droplet size of the produced emulsion.31 The droplet sizes for both formulations were below 200 nm; however, F1 produced approximately 42.13 nm with a better droplet size distribution (Figure 3), whereas F2 formed a larger droplet size of 106.00 nm (Table 2). The lower droplet size of 42.13 nm could be due to the water-soluble materials of formulation F1, which contained 20% BSO, and the system was found to be stable upon dilution. The lower polydispersity index (PDI, 0.59 for F1 and 0.48 for F2) showed in Table 2 suggests that nanodroplets were monodispersed. If the observed PDI of any SNEDDS in aqueous media is greater than 1, then it is stated as polydispersed according to the dynamic light scattering analysis (DLS).
![]() |
Table 2 Mean Droplet Size, PDI and Zeta Potential Value of the Drug-Loaded Bio-SNEDDS of Different Combinations |
![]() |
Figure 3 Droplet size distribution data for CUR and PP loaded optimized F1 and F2SNEDDS; (A) BSO: I988: TC(2:2:1)/CrRH40 [1:1 w/w] and (B) ZR: I988(7:3)/T85 [1:1 w/w], respectively. |
Droplet size plays a key role in vivo for absorption of the drug used by the oral route. The interfacial surface area increased with smaller droplet size, thus improving drug dissolution and absorption. However, it should be noted that the dispersion may be altered substantially during digestion in the presence of digestive enzymes. In addition, the droplet size, if found to be in the desirable size range (10–100 nm) could possibly enhance permeation through the cell membrane.
The zeta potential values of the SNEDDS were found to be −19.30 mV and −12.05 mV for F1 and F2, respectively (Table 2). The zeta potential of nanoparticles is generally considered the determining factor of their stability, ie, the higher the zeta potential value (negative or positive), the higher the stability of the Bio-SNEDDS formulation.28 The higher value reflected that the formulation would remain physically stable during storage. These results suggest that the higher zeta potential value of F1 is more stable than that of F2 Bio-SNEDDS and is suitable for further studies.
CUR and PP Loading (Equilibrium Solubility) into Bio-SNEDDS
Equilibrium solubility is an essential element for any drug compound because it provides the necessary information for the maximum dose that can be incorporated into a single-unit dose. In the current studies, 60% of CUR and 60% of the PP (combined) were loaded from equilibrium solubility, which was conducted and discussed in our previous studies.12 Both F1 and F2 anhydrous Bio-SNEDDS were kept for 24 h at 37°C to ensure that the solubilized state of the drug was achieved. The equilibrium solubility and 60% loading of CUR and PP in the F1 and F2 lipid-based Bio-SNEDDSs are presented in Table 3. The drug loading of CUR and PP was taken below the maximum solubility due to avoiding unwanted drug precipitation when dispersed in aqueous media.
![]() |
Table 3 Equilibrium Solubility and 60% CUR and PP Loading into Bio-SNEDDS Systems as Combined Dosage Form |
TEM Analysis
Transmission electron microscopy analyses provided information on the surface morphology of the resulting nanoemulsion and size by diluting the samples 1000 times with water and obtaining the size of the formulations. The images in Figure 4 show the spherical droplet size of F1-SNEDDS in [A] and F2-SNEDDS in [B]. The droplet sizes of the F1 SNEDDS are below 100 nm, whereas F2 showed agglomeration of the droplets.
![]() |
Figure 4 TEM images of (A) F1-Bio-SNEDDS and (B) F2-Bio-SNEDDS. |
CUR-Bio-SNEDDS Did Not Induce Toxicity in Zebrafish Embryos at Lower Concentrations
Zebrafish embryos were used in order to investigate the bioavailability and biosafety of CUR-Bio-SNEDDS. Wild-type zebrafish embryos were treated with serial dilutions of 0.1 to 100 µg/mL to assess the effect of F1 on the development of zebrafish embryos as well as to check the safety of these formulations in whole animal. One hundred percent of the zebrafish embryos (n=300±5) treated with a high dose (≥50 µg/mL) of any of the tested formulations died within a few hours of exposure. The LD50 values of CUR-Bio-SNEDDS were 30.02±0.24 and that of PP-Bio-SNEDDS was 37.68±0.36 µg/mL. However, CUR+PP treated embryos had cardiac oedema and enlarged yolk, which were not seen in F1-Bio-SNEDDS treated embryos (Figure 5).
![]() |
Figure 5 The zebrafish embryo treated with (A) control, (B) CUR and PP, and (C) optimised F1 SNEDDS formulation. |
Upon close observation, it was revealed that F1-SNEDDS treated embryos starting from 0.4 µg/mL did not have blood circulation without any other observable embryonic abnormalities shown by haemoglobin staining (Figure 6) and, video recordings (Supplementary Video 1; Supplementary Video 2). The effect of F1-Bio-SNEDDS on zebrafish haematopoiesis was also time dependent. Prolonged exposure (48 h) completely abolished haematopoiesis and resulted in no haemoglobin staining in F1- Bio-SNEDDS treated embryos (Figure 6). CUR or PP alone did not affect haematopoiesis, and there was no difference in the number of circulating blood cells in CUR and PP-treated embryos.
![]() |
Figure 6 The effect of control, pure CUR +PP powder, and F1-SNEDDS on zebrafish haematopoiesis at 48 hpf and, 96 hpf time points. “Black arrow = hemoglobin+”. |
Haematopoiesis is a normal process during embryonic development that gives rise to blood cells of different lineages. Haematopoiesis in zebrafish is similar to that in many other vertebrates and surprisingly, the developmental and genetic processes of haematopoiesis are highly conserved in zebrafish.32 Various blood cell diseases, including leukaemia, are the result of abnormalities in blood cell lineages. The zebrafish has appeared to be a prominent model for studying human leukaemia and related disorders.33
The reduced blood circulation in treated zebrafish embryos, could be due to abnormal blood vessels. Reduced blood vessels or a blockage due to clumping of blood cells could also result in defective circulation. To verify whether the F1-Bio-SNEDDS had any effect on blood vessel formation? The zebrafish transgenic line TG (Fli1: EGFP), which expresses green fluorescent protein in blood vessels, was treated. Transgenic embryos were exposed to F1-Bio-SNEDDS at the same treatment timings and concentration as in wild-type zebrafish embryos, and their effect on blood vessel formation was evaluated by observing the embryos under fluorescent microscope at 72 hpf. As shown in Figure 7, F1-SNEDDS did not affect the number or structure of blood vessels in treated embryos.
Antiproliferative Activity with THP-1 and Jurkat (Clone E6-1) (MTT Assay) Leukemia Cells
The results from this section showed that the F1- Bio-SNEDDS formulation affected the formation and development of blood cells in zebrafish embryos at concentrations in nanogram range without inducing any toxicity. This predicts possible pharmaceutical application of these formulations for the treatment of leukaemia or other haematological disorders in humans. Hence, we next tested the anticancer activity of these formulations in in vitro cell proliferation assays using two types of human haematological cancer cell lines (THP-1 and Jurkat) as well as one type of normal (non-cancer) cell line derived from Human Umbilical Vein Endothelial Cells (HUVEC). THP-1 is an immortalized monocytic cell line obtained from the blood of a patient with acute monocytic leukemia, and it is mostly utilized as a common model to estimate the modulation of monocyte and macrophage activities. It is also used to test leukemia cell lines in immunocytochemical analysis of protein–protein interactions and immunohistochemistry.34,35
Here, we investigated the antiproliferative effect of two Bio-SNEDDS formulations loaded with CUR and PP against the proliferation of HUVEC, THP-1, and Jurkat cells (Figures 8–10). Dose–response experiments (Figure 8) showed that F1 exerted the highest antiproliferative activity with an IC50 value of 18.63 µg/mL (Table 4) against THP-1 cells. We further conducted a cytotoxic evaluation for formulations against Jurkat cells. As shown in Figure 10 and Table 4, all the formulations inhibited Jurkat cells growth in a dose-dependent manner. Notably, F1 SNEDDS exhibited excellent activity (IC50 = 26.03 ± 1.5) compared to CUR and PP (Table 4).
![]() |
Table 4 Summary of IC50 Values Determined by MTT Assay |
Interestingly, we noticed that F2-SNEDDS exhibited weak cytotoxicity toward normal HUVECs in comparison to CUR (Figure 8). F1-SNEDDS also showed a less cytotoxic activity towards HUVEC cells in comparison to THP-1 and Jurkat cancer cells in terms of IC50 values (Table 4).
Discussion
Using bioactive excipients and bioactive materials in the preparation of Bio-SNEDDSs is a new concept where synergistic therapeutic benefits can be achieved. Selection of appropriate excipients, particularly oil, is the key to successful Bio-SNEDDS formulation development, which can influence biological activities along with nano droplet size and absorption of the systems. The role of BSO as a pharmaceutical excipient for the development of combined solid CUR and PP nanocarriers has been explored in our previous studies.12 In the present study, we have attempted to investigate the synergistic effects of substantially liquid Bio-SNEDDS on zebrafish embryos, normal human cells and human hematological cancer cell lines.
Two compounds that demonstrated poor water solubility, CUR and PP were developed using Bio-SNEDDS delivery systems not only to investigate their biological potentiality but also to improve their solubility and dissolution rates, which limit their oral bioavailability. The poor release (dissolution rate less than 20%) of both CUR and PP powdered drugs was reported previously and thus demand innovative formulation design. Plant derived natural oils and/or medium-chain mono, di and triglycerides (unsaturated fatty acid) are very common in pharmaceutical dosage form development for oral administration. The lipids, surfactants and cosolvents in the current Bio-SNEDDSs were suitable as vehicle for drug solubilization, aqueous dispersion and well-suited for both hard and soft gelatin capsules.
The results of Bio-SNEDDS droplet size and TEM analyses showed the effect of the uniform spherical shape of the droplets on aqueous dispersion.
WE utilised two bioactive components including black seed oil (BSO) and Zanthoxylum rhesta seed oil (ZRO) as excipients, which contained thymoquinone (TQ) and other polyphenols. TQ and PP, the active ingredients of cumin (Nigella sativa) and black pepper (Piper longum), respectively, exhibit various bioactivities including anticancer effects and the combination of TQ and PP has been previously used to target breast cancer in vitro and in vivo.29 Previous studies have shown that pure CUR and PP decreased the proliferation of various cancer cells.23 The novel combination of TQ from BSO, PP and CUR exerts its effect by inhibiting angiogenesis and inducing apoptosis as confirmed by the current studies, and demands to be used in more pre-clinical studies. These components provided additional effects along with CUR and PP in the dosage forms of F1-Bio-SNEDDS and F2-Bio-SNEDDS. Current investigations have proved the inhibitory action of CUR and PP on human hematological cancer cell lines THP1 and Jurkat cells, in a concentration-dependent manner. However, these results warrant further confirmation by rt-PCR and flow cytometry analyses using the expression of relative apoptotic markers.
We tested the toxicity of CUR formulation F1 in live zebrafish embryos. This assay has an advantage over other in vitro based studies in that the in vivo toxicity of the compound would indicate whether the compound would be suitable for further testing in clinical trials. Most anticancer drugs have been abandoned because of the induced toxicity on surrounding normal cells. Recently, efforts have been made to design better anticancer compounds that do not exhibit off-target effects. Therefore, the potential toxicity of the compounds must be assessed in suitable animals, prior to clinical trials. As embryonic development is considered to be normal process for cellular development, proliferation and cell division, zebrafish screening assays provide an excellent platform to judge whether the tested drug is safe toward normal cells. The results from the zebrafish assays in this study clearly showed that F1-Bio-SNEDDS were safe toward zebrafish embryos at low concentrations and also induced hematopoietic defects without inducing gross teratogenicity or toxicity. It is worth noting that hematopoietic defects were observed in treated embryos at concentrations in the range of 0.40 to 30.00 µg/mL; however, lethality or toxicity was observed only when zebrafish embryos were exposed to a dose over ≥50 µg/mL.
Data from this study showed that the F1-Bio-SNEDDS formulation inhibited blood cells formation in the zebrafish model. This, coupled with the inhibition of HUVEC proliferation, suggests that F1-Bio-SNEDDS might be a potential formulation to target human haematological disorders such as leukaemia, since endothelial cells are often employed in tumour microenvironment.36,37 Our findings are supported by a previous study in which indirubicin, an active ingredient in a traditional Chinese drug used to treat chronic myeloid leukaemia, was shown to inhibit HUVEC cell proliferation and angiogenesis in a zebrafish model.38 However, we did not observe the antiangiogenic effect of F1-Bio-SNEDDS in zebrafish embryos in this study. A previous study developed two SNEDDS formulations, one of α-tocopherol, resveratrol, and coenzyme Q10 and other of coumarin, and it was found that these formulations increased uptake of the drugs by at least 2-fold and significantly reduced tumour growth in female Sprague Dawley rats.39 Another study also showed that a SNEDDS formulation of deferasirox improved the drug’s cytotoxicity on the leukaemia cell line K562, and stability studies showed that the formulation might improve the drug’s oral bioavailability.40 Furthermore, the F1-Bio-SNEDDS in our study was also active against THP1 and Jurkat cell lines, inhibiting cancer cell proliferation. These data show the potential of Bio-SNEDDS in the treatment of leukaemia. Taken together, the F1 Bio-SNEDDS induced significant inhibition of blood cell proliferation both in vivo and in vitro. This indicates that this formulation might alter blood cancer cell growth and improve the bioavailability of drugs that can sustain leukaemia cancer cell killing. Additionally, a previous study showed that CUR can reverse amyloid pathology associated with neurotoxicity, which is a key component in the progression of Alzheimer’s disease.41 PP makes CUR more bioavailable; therefore, CUR is able to cross the blood–brain barrier, where it could benefit the brain and potentially prevent Alzheimer’s disease.41
Conclusion
Our studies demonstrated that F1-Bio-SNEDDSs were effectively developed which induced a significant reduction in cancer cell proliferation as well as halted haematopoiesis in the zebrafish model. These factors, when considered with their superior stability as shown by the zeta potential, may indicate an improved bioavailability that will improve the killing of leukaemia cancer cells in in vivo systems, although this remains to be extensively determined. The success of these zebrafish studies may provide the groundwork for the future development of a superior pre-clinical high-throughput model. Indeed, this model can be used to efficiently evaluate potential treatment options for leukemia subtypes by transplanting the cells of interest and then studying the efficacy and safety of combined drug regimens.
Acknowledgments
The authors would like to extend their sincere appreciation to the Researchers Supporting Project Number (RSP2023R301), King Saud University, Riyadh, Saudi Arabia.
Author Contributions
All authors made a significant contribution to the work reported, whether that is in the conception, study design, execution, acquisition of data, analysis and interpretation, or in all these areas; took part in drafting, revising or critically reviewing the article; gave final approval of the version to be published; have agreed on the journal to which the article has been submitted; and agree to be accountable for all aspects of the work.
Disclosure
Professor Mohsin Kazi reports a patent number not provided pending to Mohsin Kazi, Muhammad Farooq Khan and Mohammed S. Aldughaim. The authors declare no other conflicts of interest in this work.
References
1. Hafez Ghoran S, Calcaterra A, Abbasi M, Taktaz F, Nieselt K, Babaei E. Curcumin-based nanoformulations: a promising adjuvant towards cancer treatment. Molecules. 2022;27:16. doi:10.3390/molecules27165236
2. Alshadidi A, Shahba AA, Sales I, Rashid MA, Kazi M. Combined curcumin and lansoprazole-loaded bioactive solid self-nanoemulsifying drug delivery systems (Bio-SSNEDDS). Pharmaceutics. 2021;14(1):2. doi:10.3390/pharmaceutics14010002
3. Kazi M, Nasr A, Noman O, Alharbi A, Alqahtani MS, Alanazi FK. Development, characterization optimization, and assessment of curcumin-loaded bioactive self-nanoemulsifying formulations and their inhibitory effects on human breast cancer MCF-7 cells. Pharmaceutics. 2020;12(11):1107. doi:10.3390/pharmaceutics12111107
4. Majed Alwadei MK, Alanazi FK. Novel oral dosage regimen based on self-nanoemulsifying drug delivery systems for codelivery of phytochemicals-curcumin and thymoquinone. Saudi Pharm J. 2019;27(6):866–876.
5. Kazi M, Shariare MH, Al-bgomi M, Hussain MD, Alanazi FK. Simultaneous determination of curcumin (Cur) and thymoquinone (THQ) in lipid based self-nanoemulsifying systems and its application to the commercial product using UHPLC-UV-Vis spectrophotometer. Curr Pharm Anal. 2018;14(3):277–285. doi:10.2174/1573412913666170331114232
6. Wadhwa S, Singhal S. Bioavailability enhancement by piperine: a review. Asian J Biomed Pharm Sci. 2014;4:1.
7. Rather RA, Bhagat M. Cancer chemoprevention and piperine: molecular mechanisms and therapeutic opportunities. Front Cell Dev Biol. 2018;6. doi:10.3389/fcell.2018.00010
8. Quarti J, Torres DNM, Ferreira E, et al. Selective cytotoxicity of piperine over multidrug resistance leukemic cells. Molecules. 2021;26:4. doi:10.3390/molecules26040934
9. Manayi A, Nabavi SM, Setzer WN, Jafari S. Piperine as a potential anti-cancer agent: a review on preclinical studies. Curr Med Chem. 2018;25(37):4918–4928. doi:10.2174/0929867324666170523120656
10. Zadorozhna M, Tataranni T, Mangieri D. Piperine: role in prevention and progression of cancer. Mol Biol Rep. 2019;46(5):5617–5629. doi:10.1007/s11033-019-04927-z
11. Shoba G, Joy D, Joseph T, Majeed M, Rajendran R, Srinivas P. Influence of piperine on the pharmacokinetics of curcumin in animals and human volunteers. Planta Med. 1998;64(04):353–356. doi:10.1055/s-2006-957450
12. Kazi M, Shahba AA, Alrashoud S, Alwadei M, Sherif AY, Alanazi FK. Bioactive Self-Nanoemulsifying Drug Delivery Systems (Bio-SNEDDS) for combined oral delivery of curcumin and piperine. Molecules. 2020;25(7):1703. doi:10.3390/molecules25071703
13. Bhutani MK, Bishnoi M, Kulkarni SK. Anti-depressant like effect of curcumin and its combination with piperine in unpredictable chronic stress-induced behavioral, biochemical and neurochemical changes. Pharmacol Biochem Behav. 2009;92(1):39–43. doi:10.1016/j.pbb.2008.10.007
14. Jing L, Zon LI. Zebrafish as a model for normal and malignant hematopoiesis. Dis Model Mech. 2011;4(4):433–438. doi:10.1242/dmm.006791
15. Woo CC, Kumar AP, Sethi G, Tan KHB. Thymoquinone: potential cure for inflammatory disorders and cancer. Biochem Pharmacol. 2012;83(4):443–451. doi:10.1016/j.bcp.2011.09.029
16. Akhondian J, Kianifar H, Raoofziaee M, Moayedpour A, Toosi MB, Khajedaluee M. The effect of thymoquinone on intractable pediatric seizures (pilot study). Epilepsy Res. 2011;93(1):39–43. doi:10.1016/j.eplepsyres.2010.10.010
17. Amin F, Gilani AH, Mehmood MH, Siddiqui BS, Khatoon N. Coadministration of black seeds and turmeric shows enhanced efficacy in preventing metabolic syndrome in fructose-fed rats. J Cardiovasc Pharmacol. 2015;65(2):176–183. doi:10.1097/FJC.0000000000000179
18. Gausuzzaman SAL, Saha M, Dip SJ, et al. A QbD approach to design and to optimize the self-emulsifying resveratrol–phospholipid complex to enhance drug bioavailability through lymphatic transport. Polymers. 2022;14(15):3220. doi:10.3390/polym14153220
19. Zhang YW, Zheng Y, Zhang L, Wang Q, Zhang D. Stability of nanosuspensions in drug delivery. J Control Release. 2013;172(3):1126–1141. doi:10.1016/j.jconrel.2013.08.006
20. Alemi M, Sabouni F, Sanjarian F, Haghbeen K, Ansari S. Anti-inflammatory effect of seeds and callus of Nigella sativa L. extracts on mix glial cells with regard to their thymoquinone content. AAPS Pharm Sci Tech. 2013;14(1):160–167. doi:10.1208/s12249-012-9899-8
21. Samarakoon SR, Thabrew I, Galhena PB, De Silva D, Tennekoon KH. A comparison of the cytotoxic potential of standardized aqueous and ethanolic extracts of a polyherbal mixture comprised of Nigella sativa (seeds), Hemidesmus indicus (roots) and Smilax glabra (rhizome). Pharmacognosy Res. 2010;2(6):335–342. doi:10.4103/0974-8490.75451
22. Westerfield M. The Zebrafish Book: A Guide for the Laboratory Use of Zebrafish (Danio Rerio). University of Oregon press; 1995.
23. Nasr FA, Shahat AA, Alqahtani AS, et al. Centaurea bruguierana inhibits cell proliferation, causes cell cycle arrest, and induces apoptosis in human MCF-7 breast carcinoma cells. Mol Biol Rep. 2020;47(8):6043–6051. doi:10.1007/s11033-020-05679-x
24. Lackmann C, Santos MM, Rainieri S, et al. Novel procedures for whole organism detection and quantification of fluorescence as a measurement for oxidative stress in zebrafish (Danio rerio) larvae. Chemosphere. 2018;197:200–209. doi:10.1016/j.chemosphere.2018.01.045
25. Strähle U, Scholz S, Geisler R, et al. Zebrafish embryos as an alternative to animal experiments--a commentary on the definition of the onset of protected life stages in animal welfare regulations. Reprod Toxicol. 2012;33(2):128–132. doi:10.1016/j.reprotox.2011.06.121
26. Wadley FM. Probit analysis: a statistical treatment of the sigmoid response curve. Ann Entomol Soc Am. 1952;45(4):686. doi:10.1093/aesa/45.4.686
27. Mohsin K, Long MA, Pouton CW. Design of lipid-based formulations for oral administration of poorly water-soluble drugs: precipitation of drug after dispersion of formulations in aqueous solution. J Pharm Sci. 2009;98(10):3582–3595. doi:10.1002/jps.21659
28. Kazi M, Alhajri A, Alshehri SM, et al. Enhancing oral bioavailability of apigenin using a bioactive self-nanoemulsifying drug delivery system (Bio-SNEDDS): in vitro, in vivo and stability evaluations. Pharmaceutics. 2020;12(8):749. doi:10.3390/pharmaceutics12080749
29. Kommuru TR, Gurley B, Khan MA, Reddy IK. Self-emulsifying drug delivery systems (SEDDS) of coenzyme Q10: formulation development and bioavailability assessment. Int J Pharm. 2001;212(2):233–246. doi:10.1016/S0378-5173(00)00614-1
30. Craig DQM, Barker SA, Banning D, Booth SW. An investigation into the mechanisms of self-emulsification using particle size analysis and low frequency dielectric spectroscopy. Int J Pharm. 1995;114(1):103–110. doi:10.1016/0378-5173(94)00222-Q
31. Kazi M. Lipid‐Based Nano‐Delivery for Oral Administration of Poorly Water Soluble Drugs (PWSDs): design, Optimization and in vitro Assessment. In: Advanced Technology for Delivering Therapeutics. InTech; 2017.
32. Chen AT, Zon LI. Zebrafish blood stem cells. J Cell Biochem. 2009;108(1):35–42. doi:10.1002/jcb.22251
33. Rasighaemi P, Basheer F, Liongue C, Ward AC. Zebrafish as a model for leukemia and other hematopoietic disorders. J Hematol Oncol. 2015;8(1):29. doi:10.1186/s13045-015-0126-4
34. Chanput W, Mes JJ, Wichers HJ. THP-1 cell line: an in vitro cell model for immune modulation approach. Int Immunopharmacol. 2014;23(1):37–45. doi:10.1016/j.intimp.2014.08.002
35. Siegel RL, Miller KD, Fuchs HE, Jemal A. Cancer statistics, 2022. CA Cancer J Clin. 2022;72(1):7–33. doi:10.3322/caac.21708
36. Sobierajska K, Ciszewski WM, Sacewicz-Hofman I, Niewiarowska J. Endothelial Cells in the Tumor Microenvironment. Adv Exp Med Biol. 2020;1234:71–86.
37. Nagl L, Horvath L, Pircher A, Wolf D. Tumor Endothelial Cells (TECs) as potential immune directors of the tumor microenvironment - new findings and future perspectives. Front Cell Dev Biol. 2020;8:766. doi:10.3389/fcell.2020.00766
38. Alex D, Lam IK, Lin Z, Lee SMY. Indirubin shows anti-angiogenic activity in an in vivo zebrafish model and an in vitro HUVEC model. J Ethnopharmacol. 2010;131(2):242–247. doi:10.1016/j.jep.2010.05.016
39. Jain S, Garg T, Kushwah V, Thanki K, Agrawal AK, Dora CP. α-Tocopherol as functional excipient for resveratrol and coenzyme Q10-loaded SNEDDS for improved bioavailability and prophylaxis of breast cancer. J Drug Target. 2017;25(6):554–565. doi:10.1080/1061186X.2017.1298603
40. Alghananim A, Özalp Y, Mesut B, Serakinci N, Özsoy Y, Güngör S. A Solid Ultra Fine Self-Nanoemulsifying Drug Delivery System (S-SNEDDS) of deferasirox for improved solubility: optimization, characterization, and in vitro cytotoxicity studies. Pharmaceuticals. 2020;13(8):162. doi:10.3390/ph13080162
41. Talib WH. Regressions of breast carcinoma syngraft following treatment with piperine in combination with thymoquinone. Sci Pharm. 2017;85:3. doi:10.3390/scipharm85030027
© 2023 The Author(s). This work is published and licensed by Dove Medical Press Limited. The full terms of this license are available at https://www.dovepress.com/terms.php and incorporate the Creative Commons Attribution - Non Commercial (unported, v3.0) License.
By accessing the work you hereby accept the Terms. Non-commercial uses of the work are permitted without any further permission from Dove Medical Press Limited, provided the work is properly attributed. For permission for commercial use of this work, please see paragraphs 4.2 and 5 of our Terms.