Back to Journals » Pragmatic and Observational Research » Volume 8

Coronary stents and vascular response to implantation: literature review
Authors Brancati MF, Burzotta F, Trani C , Leonzi O, Cuccia C, Crea F
Received 15 January 2017
Accepted for publication 24 March 2017
Published 13 July 2017 Volume 2017:8 Pages 137—148
DOI https://doi.org/10.2147/POR.S132439
Checked for plagiarism Yes
Review by Single anonymous peer review
Peer reviewer comments 2
Editor who approved publication: Prof. Dr. David Price
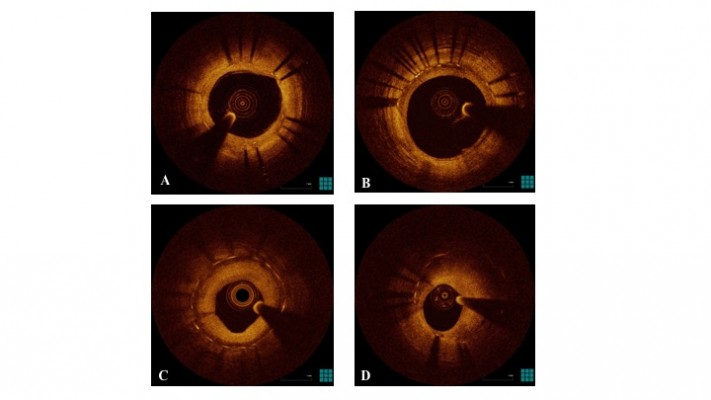
Marta Francesca Brancati,1 Francesco Burzotta,2 Carlo Trani,2 Ornella Leonzi,1 Claudio Cuccia,1 Filippo Crea2
1Cardiovascular Department, Poliambulanza Foundation Hospital, Brescia, 2Cardiovascular Department, Catholic University of the Sacred Heart, Rome, Italy
Abstract: Drug-eluting stents (DESs) have minimized the limitations of bare-metal stents (BMSs) after percutaneous coronary interventions. Nevertheless, serious concerns remain about possible late complications of stenting, such as stent thrombosis (ST) and in-stent restenosis (ISR), although the introduction of second-generation DESs seems to have softened the phenomenon, compared to the first-generation ones. ST is a potentially catastrophic event, which has been markedly reduced by optimization of stent implantation, novel stent designs, and dual antiplatelet therapy. The exact mechanism to explain its occurrence is under investigation, and, realistically, multiple factors are responsible. ISR of BMSs has been previously considered as a stable condition with an early peak (at 6 months) of intimal hyperplasia, followed by a regression period beyond 1 year. On the contrary, both clinical and histologic studies of DESs have demonstrated evidence of continuous neointimal growth during long-term follow-up, named “late catch-up” phenomenon. The acknowledgment that ISR is a relatively benign clinical condition has been recently challenged by evidences which reported that patients with ISR can experience acute coronary syndromes. Intracoronary imaging is an invasive technology that allows identifying features of atherosclerotic plaque of stent implanted and of vascular healing after stenting; it is often used to complete diagnostic coronary angiography and to drive interventional procedures. Intracoronary optical coherence tomography is currently considered a state-of-the-art imaging technique; it provides, compared to intravascular ultrasound, better resolution (at least >10 times), allowing the detailed characterization of the superficial structure of the vessel wall. Imaging studies “in vivo,” in agreement with histological findings, suggest that chronic inflammation and/or endothelial dysfunction may induce late de novo “neoatherosclerosis” inside both BMSs and DESs. So, neoatherosclerosis has become the prime suspect in the pathogenesis of late stent failure.
Keywords: coronary stents, stent thrombosis, restenosis, neoatherosclerosis
Introduction
Percutaneous coronary intervention (PCI) with stent implantation is the most widely performed procedure for the treatment of symptomatic coronary artery disease, and its technology is constantly evolving.1 Although drug-eluting stents (DESs) have minimized the limitations of bare-metal stents (BMSs), serious concerns remain about possible late complications of stenting, such as stent thrombosis (ST) and in-stent restenosis (ISR).2–5
If ST is a potentially catastrophic event, the acknowledgment that ISR is a relatively benign condition has been recently challenged by evidences of acute coronary syndromes (ACSs) in patients with ISR.4
Today, intracoronary optical coherence tomography (OCT)6–9 is considered the current state-of-the-art imaging technique, providing better resolution compared to intravascular ultrasound (IVUS). Imaging studies “in vivo,”10–12 in agreement with histological findings, showed a “new” mechanism of vascular response after stenting, de novo “neoatherosclerosis” inside both BMSs and DESs.
Coronary stents
History of coronary stents
In 1964, Charles Theodore Dotter and Melvin P Judkins described the first angioplasty. In 1978, Andreas Gruntzig performed the first balloon angioplasty (plain old balloon angioplasty); it was a revolutionary treatment, but had its own drawbacks of acute vessel closure and restenosis.13 This fueled the discovery of a coronary stent: Puel and Sigwart, in 1986, deployed the first coronary stent, able to provide a scaffold that prevented acute vessel closure and late constrictive recoil.14 Although these initial stents hampered abrupt vessel closure, they caused serious endothelial injury and inflammation. Later, two landmark trials, the Belgian Netherlands Stent trial15 and the Stent Restenosis Study,16 advocated that stenting was safe with the use of dual antiplatelet therapy (DAPT) and/or adequate technique of deployment.17,18 Following these trials, there was a phenomenal increase in the number of PCI performed.
However, the problem of iatrogenic in-stent neointimal hyperplasia after BMS placement, leading to ISR in 20%–30% of treated lesions, was soon identified. In 2001, DESs were introduced19 to minimize restenosis and requirements for reinterventions. DESs increased the confidence of cardiologists such that more and more complex lesions were treated, earlier considered to be tackled by coronary artery bypass grafting. In 2005, 80%–90% of all PCI were with DESs.
All things have a downside, and since 2005 safety concerns over “first-generation” DESs rose,20,21 and so new generation stents were developed and introduced.22 Since then, efforts to improve stent performance are fulfilled day by day, and novel, surprising technologies are continuously discovered and quickly marketed.
BMSs
BMS is a mesh-like tube of thin wire. After the first experiences with the “Wall” stent, Gianturco–Roubin stent, and the Palmaz–Schatz stent, many different BMSs are now available.
Three different designs are possible: coil, tubular mesh, and slotted tube. The coil design is characterized by metallic wire or strips formed into a circular coil shape; the tubular mesh design features wires wound together in a meshwork forming a tube; the slotted tube design is composed by tubes of metals from which a design is laser-cut. These devices differ from each other with respect to composition (stainless steel, nickel chromium alloy, cobalt chromium alloy), architectural design (different strut patterns and widths, diameters and lengths, radial strength, radiopacity), and delivery system (self-expanding or balloon expandable).
In general, new BMSs are composed by a cobalt chromium alloy, which leads to thinner struts with improved navigability, maintaining the mechanical strength.
First-generation DESs
They comprise a metallic stent platform (typically stainless steel) and are coated with a polymer that elutes antiproliferative and/or anti-inflammatory therapeutic agents.
Sirolimus-eluting stents (SESs)
Sirolimus (also known as rapamycin) was originally designed as an antimycotic agent. Its mechanism of action stems from impedance of cell cycle progression by blocking G1 to S phase transition, suppressing neointimal formation. In 2001, the “first-in-man” experience with a SES showed promising results, leading to development of Cypher stent.23 Large trials demonstrated its efficacy in preventing ISR.24
Paclitaxel-eluting stents (PESs)
Paclitaxel was initially approved for ovarian cancer, but its potent cytostatic properties – the drug stabilizes microtubules during mitosis causing cell cycle arrest and inhibition of neointimal formation – made it a compound for the Taxus Express PES. TAXUS V and VI trials demonstrated long-term efficacy of PESs in high-risk, complex coronary lesions.25,26 The subsequent TAXUS Liberté featured a more deliverable stainless steel platform.
PESs versus SESs
Conclusive evidences, derived from two systematic reviews and meta-analyses, reported superiority of SESs compared to PESs, due to diminished rates of ISR and target vessel revascularizations (TVRs), coupled with a trend toward increased acute myocardial infarction (AMI) in the PES cohort.27,28
Second-generation DESs
Second-generation devices decreased strut thickness, improved flexibility/deliverability, enhanced polymer biocompatibility/drug elution profiles, with superior re-endothelialization kinetics. In contemporary practice, they are state of the art in DES design and the predominant coronary stents implanted worldwide.
Paclitaxel
The Taxus Element was a further advancement, with a unique polymer designed to maximize early release and a novel platinum chromium strut system, providing thinner struts and enhanced radiopacity. The PERSEUS trial29 noted similar outcomes between the Element and Taxus Express up to 12 months. However, trials comparing the Taxus Element with other second-generation DESs are lacking.
Zotarolimus
The zotarolimus-eluting stent (ZES) Endeavor is based on a stronger cobalt chromium stent platform, with improved flexibility and decreased stent strut size. Zotarolimus is a sirolimus analog with similar immunosuppressant action but enhanced lipophilic properties, to enhance vessel wall localization. ZES uses a novel phosphorylcholine polymer coating designed to maximize biocompatibility and minimize inflammation. Most of the drug is eluted during the initial injury phase, thereafter allowing arterial repair. After the first ENDEAVOR trials, the subsequent ENDEAVOR III trial compared ZESs with SESs, with ZES showing greater late lumen loss and ISR but less major adverse cardiovascular events (MACE) than SESs.30 The ENDEAVOR IV trial compared ZESs with PESs and again found higher rates of ISR but fewer AMIs, ostensibly from very late ST in the ZES group.31 However, the PROTECT trial failed to demonstrate a difference in ST rates between Endeavor and Cypher stent.32
The Endeavor Resolute is a refinement of the Endeavor stent with a novel trilayered polymer. The newer Resolute Integrity (sometimes considered as a third-generation DES) is based on a new platform, with improved deliverability (Integrity BMS platform), and a novel, more biocompatible trilayered polymer, which allows suppression of the initial inflammatory response and elution of most of the drug over the next 60 days. Trial comparing the Resolute with Xience V (everolimus-eluting stent [EES]) demonstrated non-inferiority of the Resolute system in terms of death and target lesion failure.33,34
Everolimus
Everolimus, a derivative of sirolimus, is also a cell cycle inhibitor, used for the development of the Xience (Multi-link Vision BMS platform)/Promus (platinum chromium platform) EES. The SPIRIT trials35–37 demonstrated improved performance and lower MACE with Xience V compared to PESs, while the EXCELLENT trial demonstrated non-inferiority of EESs to SESs in inhibiting late loss at 9 months and clinical events at 12 months.38 Finally, Xience stent demonstrated superiority compared to BMSs also in the setting of ST-elevation myocardial infarction (MI).39
Novel technologies
Endothelial progenitor cells (EPCs)
EPCs are a subset of circulating cells implicated in vascular homeostasis and endothelial repair. Enhancement of EPCs at sites of vascular injury would promote early re-endothelialization, potentially reducing the risk of ST. The first attempt of application of EPC biology in the field of stent design was the CD34 antibody-coated Genous stent, capable of binding circulating EPCs by their hematopoietic marker, to enhance re-endothelialization. Despite initial encouraging studies, recent evidences suggested high TVR rates.40
Bioresorbable polymer stents and polymer-free stents
Considering the potential deleterious effects of polymer-induced delayed healing, which was correlated to the risk of ST, bioresorbable polymers providing the benefits of a DES, avoiding long-term concerns on persistence of polymer, were explored. To date, different bioresorbable systems are approved for use (eg, Nobori and Biomatrix, biolimus-eluting stent, Synergy, EES, Ultimaster, SES), although literature on long-term outcomes supporting them is limited.41
Polymer-free stents are theoretically promising, especially in patients at high bleeding risk.42
Bioresorbable scaffolds
Bioresorbable materials offer the theoretical advantage of providing mechanical support initially, when elastic recoil is a concern, and reducing long-term risks associated with existing metallic struts. Novel technologies led to the development of a lactic acid-based polymer (poly-l-lactic acid [PLLA]), but numerous stenting systems are under development, though identifying the ideal balance between drug elution and degradation dynamics remains a challenge. The ABSORB trial demonstrated safety and efficacy of the everolimus-eluting PLLA scaffold.43 The second-generation Absorb scaffold revision is an evolution of the previous one with good 2-year follow-up.44 The ongoing ABSORB II trial, the first randomized trial comparing the Absorb scaffold with the Xience Prime stent, should give further data, and the first available results are promising.45 However, ideal setting of coronary lesions, optimal technique of implantation, and safety profiles need to be better clarified.
ST
Definition of ST
The Academic Research Consortium definitions46 standardize ST diagnosis (Table 1).
![]() ![]() | Table 1 Stent thrombosis definitions and classification according to ARC Note: Data from Cutlip et al.46 Abbreviation: ARC, Academic Research Consortium. |
Clinical presentation of ST
Thrombosis of both BMSs and DESs has adverse clinical outcome. In a registry of patients who underwent DES implantation,47 24% of ST cases resulted in death, 60% in nonfatal MI, and 7% in unstable angina. PCI for emergent ST is often suboptimal, and ST recurs in 12% of cases.48
Late ST has a potential adverse clinical outcome. In the BASKET-LATE study, between 6 and 18 months from stenting, rates of cardiac death and nonfatal MI were higher in the DES than in the BMS group (4.9% vs 1.3%, respectively).20 A meta-analysis of nine trials, in which 5,261 patients were randomized to SESs, PESs, or BMSs, reported, over 4 years of follow-up, increased rates of very late ST for both SESs (0.6% vs 0%, p=0.025) and PESs (0.7% vs 0.2%, p=0.028) compared to BMSs.49 Conversely, in a meta-analysis including 5,108 patients,21 a 60% relative increase in death or MI (p=0.03) for SESs compared to BMSs was reported, whereas PESs were associated with a nonsignificant increase of 15% (follow-up over 9 months to 3 years).
Incidence of ST
First-generation DESs
Many registries, randomized trials, and meta-analyses have investigated the relative risk for ST after BMS and DES implantation and reported conflicting results. In a registry of 6,906 patients who received BMS or DES, there was no difference in clinical outcomes or ST rate over 1-year follow-up.48 In another registry of 8,146 patients, a persistent excess ST risk of 0.6%/year was found compared with BMSs.49 A meta-analysis from trials comparing SESs or PESs versus BMSs suggested an increased risk of mortality and MI with first-generation DESs compared to BMSs,21 while another meta-analysis in which 4,545 patients were randomized to SESs or PESs versus BMSs revealed no difference in rates of ST over 4 years of follow-up.50 Additional real-world studies showed an increased risk of late ST and MI in patients treated with first-generation DESs after discontinuation of DAPT.51
In view of the conflicting evidences, several pooled analyses and meta-analyses52 collectively established no significant differences in the risk of death or MI between first-generation DESs and BMSs, but an increased risk of very late ST with both SESs and PESs compared to BMSs. To review the available evidence, the Food and Drug Administration (FDA) assigned an expert panel53 that released a statement recognizing first-generation DESs as effective for on-label indications, acknowledging a small but significant increased risk of very late ST. As a consequence, FDA and societies recommended lengthening the DAPT period to 1 year, although little data support this statement.
Second-generation DESs
Second-generation DESs have been developed with advanced design features, as previously described. CoCr-EESs have undergone the most extensive clinical investigation. In a meta-analysis by Baber et al,54 which included 17,101 patients, CoCr-EESs significantly reduced definite/probable ST and MI compared to PESs, SESs, and ZES after 21 months. Finally, Palmerini et al, in a meta-analysis with 16,775 patients, showed significant reduction of early, late, 1-year, and 2-year definite ST with CoCr-EESs compared to other pooled DESs.55 Real-world studies confirmed the reduced risk of ST with CoCr-EESs compared to first-generation DESs.56
Re-ZES has been compared with CoCr-EESs in the RESOLUTE-AC and TWENTE trials.33,57 No significant differences in rates of death, MI, or definite ST were apparent between the two stents.
In a network meta-analysis including 49 randomized controlled trials with 50,844 patients,58 CoCr-EESs were associated with significantly lower rates of definite ST than BMSs, a result not observed with other DESs; the reduction was apparent not only early and at 30 days (odds ratio [OR] 0.21, 95% confidence interval [CI] 0.11–0.42) but also at 1 year (OR 0.27, 95% CI 0.08–0.74) and at 2 years (OR 0.35, 95% CI 0.17–0.69). CoCr-EESs were also associated with lower rates of ST compared to PESs, SESs, and ZESs at 1 year.
Mechanisms of ST
Early versus late/very late ST
Early ST is related to different factors. Underlying plaque morphology and thrombus burden seem to influence the outcome after PCI;59 deeper struts penetration by necrotic core (NC) prolapse, long medial tear within the stent, suboptimal stenting with residual edge dissections or significant edge stenosis, incomplete apposition, and incomplete expansion of implanted stent may increase the risk of ST.60 Therapeutic regimen of antiplatelet drugs does not substantially influence incidence of early ST: in a randomized trial comparing BMSs with DESs, the rates of acute and subacute ST during DAPT were similar (<1%).61 So, early ST seems to be primarily related to underlying treated lesions and to procedural factors.
Today, particular attention is focused on late/very late ST. If procedural and technical factors seem to play a major role in the occurrence of acute and subacute ST, mechanisms of delayed thrombotic events seem to be more complex. It has been suggested that some patient characteristics may be risk factors for late and very late ST: diabetes, ACS during the original procedure, renal failure, advanced age, reduced ejection fraction, major cardiac adverse events within 30 days from the original procedure. Procedural variables, such as small vessel size, bifurcations, multivessel disease, calcification, total occlusions, long stents, seem to be associated with the risk of late ST, both for BMSs and for DESs.62,63 Inadequate response to antiplatelet therapy is a major risk factor for late DES thrombosis.51 This response might be due to patient noncompliance, underdosing, drug interactions, comorbidities affecting drug response, genetic polymorphism at the receptor level (particularly for clopidogrel resistance), upregulation of other platelet activation pathways. In-stent neoatherosclerosis is considered an important mechanism of late stent failure, including late ST64 (“In-stent neoatherosclerosis” section). The intact endothelium separates the thrombogenic vessel wall and stent struts from the blood stream and secretes antithrombotic and vasodilator substances. DESs expose the vessel wall to antiproliferative drugs and drug-eluting platforms, with variable effects on endothelial healing and function, with late thrombotic risk.65 Pathological studies showed that the durable polymer of first-generation DESs could result in chronic inflammation, with chronic fibrin deposition, poor endothelial healing, and consequent increased thrombotic risk.3 Late hypersensitivity reactions to DESs seem to be another mechanism contributing to ST. Virmani et al66 reported autopsy findings after late ST, showing aneurysmal dilation of stented segment with a localized hypersensitivity reaction consisting of T lymphocytes and eosinophils; these findings likely reflect an effect of the non-erodable polymer.67 Malapposition of the stent might result from suboptimal stent expansion or develop months after PCI. Although procedural malapposition is a risk factor for acute and subacute ST, the clinical significance of acquired stent malapposition, which may depend from positive artery remodeling or from drug-induced delayed healing, is controversial.68
Mechanisms potentially explaining the reduced ST with second-generation DESs
Protective effect of second-generation DESs likely includes more rapid and complete endothelialization as well as differences in stent alloy and architecture, strut thickness, polymer characteristics, and antiproliferative drug type, dose, and kinetics.
Relative to CoCr-EESs, the thin (81 µm) cobalt chromium stent struts, the thromboresistant fluoropolymer, the low polymer, and drug load may contribute to the low rate of ST. Experimental studies demonstrated that fluoropolymer-coated stents had significantly lower thrombosis and platelet deposition than bare-metal counterparts.69 Whether similar properties are shared by other second-generation DESs deserves future investigations.
ISR
Incidence and timing of ISR
Coronary stents improved procedural success of coronary interventions, compared to conventional percutaneous transluminal coronary angioplasty (PTCA), which was burdened by mechanical complications (vessel occlusion, dissection, etc) and high rate of restenosis (up to 40%–50% of cases). By the end of the 1990s, nearly 70% of PCIs were performed with a BMS implantation.70
However, despite advances in technologies, technique, and medical therapy, the risk of restenosis after BMS implantation is ~20%, with rates of >40% in particular subsets.71 In general, it was demonstrated by clinical studies that the time course of restenosis after BMS implantation, similar to that observed for conventional PTCA, shows a peak at 3–6 months, and a regression period beyond 1 year.72
DESs have further decreased the incidence of ISR,73 although this reduction depends on the angiographic and clinical setting. The polymeric coating on DESs releases anti-inflammatory and antiproliferative agents, which inhibit neointimal formation, and delay the vessel reparative process by months to years.74 Continuous neointimal growth during long-term follow-up after DES implantation, named “late catch-up” phenomenon, was observed by clinical and histologic studies.75
Pathophysiology of ISR
Vascular injury occurring during PCI produces, over a relatively short time (weeks to months), a complex inflammatory and reparative process, resulting in endothelialization and neointimal coverage. According to histopathologic observations, neointimal hyperplasia after stent implantation (both BMSs and DESs) is mainly composed of proliferative smooth muscle cells in a proteoglycans-rich extracellular matrix.70
So, neointimal hyperplasia represents a reparative process, involving coagulation and inflammatory factors and cells that induce smooth muscle cell proliferation and extracellular matrix formation. Immediately after PCI, platelets and fibrin deposit on the vessel wall and recruit leukocytes through a cascade of cell adhesion molecules. Rolling leukocytes attach to adherent platelets through the interaction between the leukocyte integrin Mac-1 (CD11b/CD18) and platelet glycoprotein Ibα 53 or fibrinogen bound to the platelet glycoprotein IIb/IIIa.76,77
According to emerging data, bone marrow-derived progenitor cells are involved in vascular responses and repair processes. EPCs mobilized from bone marrow into peripheral blood promote endothelial regeneration and postnatal neovascularization. It seems that bone marrow smooth muscle progenitor cells (SMPCs) migrate to the sites of vascular injury, contributing to neointimal proliferation.78 Previously, CD34-positive cells were believed to be the committed population of EPCs; further study demonstrated that the CD34 surface antigen actually identifies undifferentiated bone marrow-derived stem cells that have the ability to differentiate into EPC and SMPCs. Transdifferentiation of CD34-positive cells into EPC or SMPC lineages depends on the local environment; ischemic conditions induce differentiation toward EPC phenotypes in order to promote re-endothelialization, while inflammatory conditions induce differentiation toward SMPC phenotypes in order to promote neointimal proliferation.79
Predictors of ISR
Principal predictors of ISR are summarized in Table 2.
![]() ![]() | Table 2 Predictors of ISR Abbreviation: ISR, in-stent restenosis. |
Patient factors
Diabetes mellitus amplifies the risk of ISR by 30%–50% after BMS implantation,80 and the higher incidence of restenosis in diabetic patients, compared to nondiabetic ones, persists also in the DES era. The mechanism underlying this observation is probably multifactorial, involving both systemic (eg, variability in the inflammatory response) and anatomic (eg, smaller diameter vessels, longer lesions, diffuse disease, etc) factors, which independently increase the risk of ISR.70
Anatomic factors
Vessel diameter and lesion length independently impact the incidence of ISR, and smaller diameter/longer lesions significantly increase restenosis rates compared to larger diameter/shorter lesions.71
Procedural variables
First-generation stent platforms showed thicker stent struts, which were burdened by higher rates of ISR when compared to second-generation stent platforms with thinner struts.
Moreover, incidence of restenosis is associated with the stent length, nearly doubling for stent lengths >35 mm compared to those <20 mm. Final stent minimal lumen diameter also plays a major role: smaller final minimal lumen diameter predicts a significantly increased risk of restenosis.81,82
In-stent neoatherosclerosis
Late restenosis
Traditionally, intimal hyperplasia after BMS implantation has been considered stable, with an early peak between 6 months and 1 year and a late quiescent period thereafter. An early peak of intimal growth, followed by intimal regression with luminal enlargement some years after stent implantation was previously reported;71 maturation of smooth muscle cells with modification of extracellular matrix was postulated as the possible mechanisms of late neointimal regression.83 However, further long-term follow-up studies demonstrated a triphasic response after BMS placement, with an early restenosis, an intermediate regression, and a late-luminal re-narrowing.84
In the DES era, late neointimal growth was demonstrated, initially in animal models, after SES or PES implantation.85 Several IVUS studies showed early attenuation of intimal growth, followed by late catch-up over time after SES or PES implantation, probably due to persistent inflammatory process.86
“De novo” neoatherosclerosis
Histologic features and timing
About one-third of patients with ISR of BMSs presents with ACS, in spite of the “stability” traditionally attributed to ISR.4
There is emerging evidence that chronic inflammation and/or incompetent endothelial function induce late de novo neoatherosclerosis inside both BMSs and DESs (mainly first-generation DESs), which may be an important mechanism of late ISR or late ST. Inoue et al87 reported histologic findings of autopsied samples after implantation of Palmaz–Schatz coronary stents, suggesting the possibility that peri-strut inflammation might accelerate new indolent atherosclerotic changes within the stents. Other studies10 demonstrated that restenotic tissues inside BMSs, beyond 5 years, were composed of newly developed atherosclerosis regardless of the presence of peri-strut inflammation; samples from the cases presented with ACS exhibited typical histologic morphologies of vulnerable plaque in native coronary arteries, with foamy macrophages and cholesterol crystals. Moreover, when comparing BMSs and DESs, a significant difference in the timing of neoatherosclerosis development was noted.11,12 The earliest atherosclerotic change with foamy macrophage infiltration began at 4 months after SES implantation, whereas the same change in BMS lesions occurred beyond 2 years and remained a rare finding until 4 years. In addition, unstable lesions like thin-cap fibroatheromas (TCFA) or intimal rupture had shorter timing of development from stenting for DESs compared to BMSs. So, neoatherosclerosis in first-generation DESs seems more frequent and occurs earlier than in BMSs, likely from different pathogenesis.
The effects of second-generation DESs or developing DESs have yet to be investigated; although some available observations on second-generation DESs88 suggest less inflammation but similar rate of neoatherosclerosis compared to first-generation ones, further studies are warranted.
Pathophysiology
The cellular mechanisms involved in neoatherosclerosis focus on the endothelium. This is not surprising, as early studies in the era of balloon angioplasty demonstrated that regenerated endothelial cells are structurally and functionally abnormal.89 Early pathological studies of BMSs implanted for 2–7 years revealed chronic inflammation, neointimal microvessels, extracellular matrix collagen deposition, and matrix metalloproteinase activity.87 It should be expected that after stent placement the regenerated endothelium would be functionally incompetent, with poor atheroprotective features and incomplete cell–cell junctions which are permissive for lipid or inflammatory cell migration into the vessel wall. Shear stress modification, due to the presence of stent, induces endothelial cells to express vascular cell adhesion molecule 1 and intercellular adhesion molecule 1 near stent struts,90 allowing the adhesion and diapedesis of leukocytes into the subendothelium, where they become foam cells. Moreover DESs, releasing antiproliferative drugs, promote chronic inflammation and recruitment of lymphocytes and monocytes and may determine a greater and earlier endothelial dysfunction, with lower production of nitric oxide and antithrombotic factors and gap junction malfunction.
In-stent neoatherosclerosis as a predictor of late stent failure
The extensive data as reviewed in the preceding text support the importance of in-stent neoatherosclerosis as a mechanism of late ST after either BMS or DES implantation. Although incomplete endothelialization with uncovered struts remain the primary cause of DES thrombosis, advanced neoatherosclerosis with neointimal rupture is also suggested as another contributing factor to late and very late thrombotic events.12
Angioscopic and IVUS data91 reported intramural thrombi in newly formed yellow neointima, as well as neoatherosclerotic progression with intimal rupture.
It is clear that neoatherosclerosis plays a role as an important indicator of late ST and late restenosis in both BMSs and DESs, but more importantly in DESs, as it occurs much earlier. However, the lack of knowledge regarding the exact mechanisms underlying this phenomenon warrants further investigation on this topic.
Intracoronary imaging for the evaluation of coronary stent healing
IVUS imaging modality
Technical aspects
IVUS allows the application of ultrasound technology to coronary imaging. This technique employs a special catheter with a miniaturized ultrasound probe attached to its distal end and a computerized ultrasound equipment attached to its proximal end. The 6-F compatible mechanical systems offer more uniform pullback and greater resolution due to the higher ultrasound frequency (40 MHz). The solid-state phased array transducer has 64 stationary transducer elements around the tip that image at 20 MHz, and it is commercially available as 5-F compatible.92 High-frequency 60 MHz IVUS catheters have comparable resolution to OCT and may play a comparable role to OCT in the evaluation of coronary lesions.
Virtual histology (VH) IVUS
IVUS with VH employs spectral analysis of the radiofrequency ultrasound backscatter signals to overcome the limitations of grayscale IVUS and identify the components of the atherosclerotic plaque (or of the restenotic tissue).
To simplify image interpretation, tissue components are grouped into four types, displayed on the image as different color pixels:
- fibrous = green;
- fibrofatty = light green;
- NC = red;
- dense calcium = white.
The technique is based on advanced radiofrequency analysis of reflected ultrasound signals and displays the reconstructed color-coded tissue map of plaque composition superimposed on cross-sectional images of the coronary artery, obtained by grayscale IVUS.93
IVUS and VH-IVUS in the evaluation of vessel response after stent implantation
Although it is difficult for IVUS to classify neointimal tissue because of the signal interference from metal struts, there are several reports attempting discrimination of neointimal tissues by IVUS, demonstrating plaque rupture and a flap-like dissection inside a restenotic stent.
Kang et al94 reported findings from 70 DES-ISR and 47 BMS-ISR lesions with intimal hyperplasia in more than 50% of the stent area by VH-IVUS.
The region of interest (ROI) was placed between the luminal border and the inner border of the struts, and tissue composition was represented as percentages of intimal area.
In both DES and BMS groups, NC and dense calcium suggesting neoatherosclerosis were greater especially in the lesions with longer implant duration. However, even though VH-IVUS is histologically validated in the assessment of the compositions of naïve atherosclerotic plaques with high accuracy (94% for NC and 99% for dense calcium), the methodology needs to be revisited for the evaluation of neointimal characteristics.
OCT imaging modality
Technical aspects
OCT is a near-infrared light-based imaging modality with ultrahigh resolution (10–20 µm, 10-fold higher than IVUS).
The light source used for OCT imaging is in the near-infrared range, around 1,300 nm wavelength, selected as a compromise to achieve both penetration and delineation of vascular structures.
Intravascular OCT requires a blood-free field lasting several seconds to allow imaging. Early commercially available versions of the technology used time domain (TD) detection, which achieved the blood-free field either by injecting continuous saline/contrast flushes through the guiding or delivery catheters, or by using a proximal balloon occlusion of the vessel with distal saline/contrast injection.95 Currently available systems use a higher speed method, the frequency or Fourier domain (FD) detection, which measures all echoes of light from different depths simultaneously, rather than sequentially as in TD-OCT, with imaging speeds that are >10 times faster than TD-OCT.96 So, image acquisition is possible during a contrast or saline flush lasting 3–5 seconds. FD-OCT systems do not require proximal occlusion; the coronary vessel is transiently rendered free of blood by a bolus injection of saline, contrast, or other solution. The monorail rapid exchange catheter is compatible with 6-F guiding catheters and can be conveniently incorporated into most interventional procedures.
Expert review documents defined the methodology and current clinical application of OCT.6,7
“Quantitative” OCT tissue properties analysis
Dedicated software packages provide tissue analysis tools that are able to quantitatively assess the optical properties of OCT images. Till now, they are mainly employed for research use, and few studies are available.
The signal intensity from a tissue region is determined by two factors: the incident light intensity and the backscattering ability of the region.
Quantitative measurements of tissue evaluate the following parameters:
- attenuation (the gradual loss in intensity of the ROI);
- backscatter (the diffuse reflection due to nonuniformities in the ROI);
- mean intensity (the brightness of the signal);
- variance (a statistical measure of the ROI homogeneity).
Neointimal patterns according to OCT assessment
According to previous descriptions,97 OCT is able to distinguish the following neointimal patterns (Figure 1):
- homogeneous neointima, a uniform signal-rich band without focal variation or attenuation;
- heterogeneous neointima, focally changing optical properties and various backscattering patterns;
- layered neointima, layers with different optical properties, an adluminal high scattering layer and abluminal low scattering layer;
- neoatherosclerotic neointima, containing at least one of the following features: lipid-laden neointima, neointima with calcification, TCFA-like neointima, and neointimal rupture.
![]() ![]() | Figure 1 OCT patterns of neointima. Notes: (A) Homogeneous pattern; (B) heterogeneous pattern; (C) layered pattern; (D) neoatherosclerosis. Abbreviation: OCT, optical coherence tomography. |
Lipid-laden neointima is defined as a signal-poor region with diffuse borders, masking stent struts behind; it is different from neointima with calcification, that is a signal-poor region with sharp borders. A TCFA-like neointima is defined as neointima with a fibrous cap thickness at the thinnest part ≤65 μm and an angle of lipid-laden neointima ≥180°. Neointimal rupture is a break in the fibrous cap, connecting the lumen with the underlying lipid pool. Intraluminal material is visible material inside the vessel lumen. Microvessels are well-delineated low backscattering structures <200 μm in diameter showing a trajectory within the vessel.
OCT in the evaluation of vessel response after stent implantation
Due to its excellent resolution, OCT was recently developed as a new intravascular imaging technique to assess stent morphology and vessel response after stent implantation in the catheterization laboratory.
OCT provides more detailed information, compared to IVUS, on acute findings, such as stent edge dissection, tissue protrusion, and incomplete stent apposition immediately after stent implantation.98
OCT demonstrated its potential capacity to accurately characterize or evaluate vascular responses after months or years since stent implantation. Habara et al99 examined restenotic lesions >5 years after BMS implantation and found a high incidence (90.7%) of possible neoatherosclerotic change, defined as OCT appearance with low-intensity areas, whereas lesions <1 year after BMS implantation showed only 17.9% incidence of neoatherosclerosis. Neointimal disruption, which has an analogous morphology of ruptured TCFA in a native coronary artery, occurred more frequently in >5-year lesions (18.6%) than in <1-year lesions (0%). Takano et al100 described neointimal OCT characteristics of BMSs in early (<6 months) and late phases (≥5 years). In the early phase, neointima exhibited a homogeneous OCT appearance, without lipid-laden intima. Conversely, in the late phase, lipid-laden intima, intimal disruption, and luminal thrombus formation were more frequently observed compared to the early phase (67% vs 0%, 38% vs 0%, and 52% vs 5%, respectively; all p<0.05).
A recent OCT analysis in 50 patients with DES-ISR (median follow-up period of 32.2 months) demonstrated that 52% of overall lesions had at least 1 TCFA, 58% in-stent neointimal rupture, and 58% intraluminal thrombi.101 Unstable clinical presentation was associated with unstable OCT neointimal findings, including TCFA, neointimal rupture, and thrombus. DESs ≥20 months postimplantation had a higher incidence of TCFA and red thrombi (27% vs 0%, p=0.007).
So, OCT contributed to clarify that neoatherosclerosis is an important mechanism of late stent failure, with (often) unstable clinical presentation; OCT imaging was also important to understand that neoatherosclerotic process undergoes during an extended follow-up period, but earlier for DESs (mainly first-generation ones) than for BMSs.
Summary
Second-generation DESs have minimized the limitations of BMSs and first-generation DESs after PCIs. However, serious concerns remain about late complications of stenting, such as ST and ISR.
ST is a potentially catastrophic event. Multiple factors are responsible for this phenomenon, such as suboptimal stent implantation, complex lesions, and low compliance to DAPT.
ISR of BMSs has been previously considered as a stable condition with an early peak, followed by a regression period beyond 1 year. The acknowledgment that this condition is relatively benign has been recently challenged by clinical evidences of ACSs associated with angiographic documentation of ISR. Histologic studies and intracoronary imaging (which allows a detailed characterization of vessel wall) suggest that chronic inflammation and/or endothelial dysfunction may induce late de novo “neoatherosclerosis” inside both BMSs and DESs. Neoatherosclerosis plays an important role in the pathogenesis of late stent failure.
Disclosure
The authors report no conflicts of interest in this work.
References
Serruys PW, Rutherford JD. The birth, and evolution, of percutaneous coronary interventions: a conversation with Patrick Serruys, MD, PhD. Circulation. 2016;134:97–100. | ||
Liistro F, Stankovic G, Di Mario C. First clinical experience with a paclitaxel derivate-eluting polymer stent system implantation for in-stent restenosis: immediate and long-term clinical and angiographic outcome. Circulation. 2002;105:1883–1886. | ||
Finn AV, Nakazawa G, Joner M. Vascular responses to drug eluting stents: importance of delayed healing. Arterioscler Thromb Vasc Biol. 2007;27:1500–1510. | ||
Chen MS, John JM, Chew DP, Lee DS, Ellis SG, Bhatt DL. Bare metal stent restenosis is not a benign clinical entity. Am Heart J. 2006; 151:1260–1264. | ||
Jaffe R, Strauss BH. Late and very late thrombosis of drug-eluting stents: evolving concepts and perspectives. J Am Coll Cardiol. 2007;50:119–127. | ||
Prati F, Regar E, Mintz GS, et al. Expert review document on methodology, terminology, and clinical applications of optical coherence tomography: physical principles, methodology of image acquisition, and clinical application for assessment of coronary arteries and atherosclerosis. Eur Heart J. 2010;31:401–415. | ||
Prati F, Guagliumi G, Mintz GS, et al. Expert review document part 2: methodology, terminology, and clinical applications of optical coherence tomography for the assessment of interventional procedures. Eur Heart J. 2012;33:2513–2520. | ||
Tearney GJ, Regar E, Akasaka T, et al. Consensus standards for acquisition, measurement, and reporting of intravascular optical coherence tomography studies: a report from the international working group for intravascular optical coherence tomography standardization and validation. J Am Coll Cardiol. 2012;59:1058–1072. | ||
Di Vito L, Yoon JH, Kato K, et al. Comprehensive overview of definitions for optical coherence tomography-based plaque and stent analyses. Coron Artery Dis. 2014;25:172–185. | ||
Hasegawa K, Tamai H, Kyo E, et al. Histopathological findings of new in-stent lesions developed beyond five years. Catheter Cardiovasc Interv. 2006;68:554–558. | ||
Nakazawa G, Vorpahl M, Finn AV, Narula J, Virmani R. One step forward and two steps back with drug-eluting stents: from preventing restenosis to causing late thrombosis and nouveau atherosclerosis. JACC Cardiovasc Imaging. 2009;2(5):625–628. | ||
Nakazawa G, Otsuka F, Nakano M, et al. The pathology of neoatherosclerosis in human coronary implants bare-metal and drug-eluting stents. J Am Coll Cardiol. 2011;57:1314–1322. | ||
Gruntzig AR, Senning A, Siegenthaler WE. Nonoperative dilatation of coronary-artery stenosis: percutaneous transluminal coronary angioplasty. N Engl J Med. 1979;301:61–68. | ||
Sigwart U, Puel J, Mirkovitch V, Joffre F, Kappenberger L. Intravascular stents to prevent occlusion and restenosis after transluminal angioplasty. N Engl J Med. 1987;316:701–706. | ||
Serruys PW, de Jaegere P, Kiemeneij F, et al. A comparison of balloon-expandable-stent implantation with balloon angioplasty in patients with coronary artery disease. N Engl J Med. 1994;331:489–495. | ||
Fischman DL, Leon MB, Baim DS, et al. A randomized comparison of coronary-stent placement and balloon angioplasty in the treatment of coronary artery disease. N Engl J Med. 1994;331:496–501. | ||
Schomig A, Neumann FJ, Kastrati A, et al. A randomized comparison of antiplatelet and anticoagulant therapy after the placement of coronary-artery stents. N Engl J Med. 1996;334:1084–1089. | ||
Colombo A, Hall P, Nakamura S, et al. Intracoronary stenting without anticoagulation accomplished with intravascular ultrasound guidance. Circulation. 1995;91:1676–1688. | ||
Sousa JE, Costa MA, Abizaid A, et al. Lack of neointimal proliferation after implantation of sirolimus-coated stents in human coronary arteries: a quantitative coronary angiography and three-dimensional intravascular ultrasound study. Circulation. 2001;103:192–195. | ||
Pfisterer M, Brunner-La Rocca HP, Buser PT. Late clinical events after clopidogrel discontinuation may limit the benefit of drug-eluting stents: an observational study of drug-eluting versus bare-metal stents. J Am Coll Cardiol. 2006;48:2584–2591. | ||
Camenzind E, Steg PG, Wijns W. Stent thrombosis late after implantation of first-generation drug-eluting stents: a cause for concern. Circulation. 2007;115:1440–1455. | ||
Kereiakes DJ, Cox DA, Hermiller JB, et al. Usefulness of a cobalt chromium coronary stent alloy. Am J Cardiol. 2003;92:463–466. | ||
Sousa JE, Costa MA, Abizaid AC, et al. Sustained suppression of neointimal proliferation by sirolimus-eluting stents: one-year angiographic and intravascular ultrasound follow-up. Circulation. 2001;104:2007–2011. | ||
Morice MC, Serruys PW, Sousa JE, et al. A randomized comparison of a sirolimus-eluting stent with a standard stent for coronary revascularization. N Engl J Med. 2002;346:1773–1780. | ||
Stone GW, Ellis SG, Cannon L, et al. Comparison of a polymer-based paclitaxel-eluting stent with a bare metal stent in patients with complex coronary artery disease: a randomized controlled trial. JAMA. 2005;294:1215–1223. | ||
Dawkins KD, Grube E, Guagliumi G, et al. Clinical efficacy of polymer-based paclitaxel-eluting stents in the treatment of complex, long coronary artery lesions from a multicenter, randomized trial: support for the use of drug-eluting stents in contemporary clinical practice. Circulation. 2005;112:3306–3313. | ||
Kastrati A, Dibra A, Eberle S, et al. Sirolimus-eluting stents vs paclitaxel-eluting stents in patients with coronary artery disease: meta-analysis of randomized trials. JAMA. 2005;294:819–825. | ||
Schomig A, Dibra A, Windecker S, et al. A meta-analysis of 16 randomized trials of sirolimus-eluting stents versus paclitaxel-eluting stents in patients with coronary artery disease. J Am Coll Cardiol. 2007;50:1373–1380. | ||
Kereiakes DJ, Cannon LA, Feldman RL, et al. Clinical and angiographic outcomes after treatment of de novo coronary stenoses with a novel platinum chromium thin-strut stent: primary results of the PERSEUS (prospective evaluation in a randomized trial of the safety and efficacy of the use of the TAXUS Element paclitaxel-eluting coronary stent system) trial. J Am Coll Cardiol. 2010;56:264–271. | ||
Kandzari DE, Leon MB, Popma JJ, et al. Comparison of zotarolimus-eluting and sirolimus-eluting stents in patients with native coronary artery disease. A randomized controlled trial. J Am Coll Cardiol. 2006;48:2440–2447. | ||
Leon MB, Nikolsky E, Cutlip DE, et al. Improved late clinical safety with zotarolimus-eluting stents compared with paclitaxel-eluting stents in patients with de novo coronary lesions: 3-year follow-up from the ENDEAVOR IV (randomized comparison of zotarolimus- and paclitaxel-eluting stents in patients with coronary artery disease) trial. JACC Cardiovasc Interv. 2010;3:1043–1050. | ||
Camenzind E, Wijns W, Mauri L, et al. Stent thrombosis and major clinical events at 3 years after zotarolimus-eluting or sirolimus-eluting coronary stent implantation: a randomised, multicentre, open-label, controlled trial. Lancet. 2012;380:1396–1405. | ||
Taniwaki M, Stefanini GG, Silber S, et al. 4-Year clinical outcomes and predictors of repeat revascularization in patients treated with new-generation drug-eluting stents: a report from the RESOLUTE All-Comers trial (a randomized comparison of a zotarolimus-eluting stent with an everolimus-eluting stent for percutaneous coronary intervention). J Am Coll Cardiol. 2014;63:1617–1625. | ||
Serruys PW, Silber G, Garg S, et al. Comparison of zotarolimus-eluting and everolimus-eluting coronary stents. N Engl J Med. 2010; 363:136–146. | ||
Serruys PW, Ong AT, Piek JJ, et al. A randomized comparison of a durable polymer everolimus-eluting stent with a bare metal coronary stent: the SPIRIT first trial. Eurointervention. 2005;1:58–65. | ||
Serruys PW, Ruygrok P, Neuzner J, et al. A randomised comparison of an everolimus-eluting coronary stent with a paclitaxel-eluting coronary stent: the SPIRIT II trial. Eurointervention. 2006;2:286–294. | ||
Stone GW, Midei M, Newman W, et al. Comparison of an everolimus-eluting stent and a paclitaxel-eluting stent in patients with coronary artery disease: a randomized trial. JAMA. 2008;299:1903–1913. | ||
Park KW, Chae IH, Lim DS, et al . Everolimus-eluting versus sirolimus-eluting stents in patients undergoing percutaneous coronary intervention: the EXCELLENT (efficacy of Xience/Primus versus Cypher to reduce late loss after stenting) randomized trial. J Am Coll Cardiol. 2011;58:1844–1854. | ||
Sabate M, Cequier A, Iñiguez A, et al. Everolimus-eluting stent versus bare-metal stent in ST-segment elevation myocardial infarction (EXAMINATION): 1 year results of a randomized controlled trial. Lancet. 2012;380:1482–1490. | ||
Beijk MA, Klomp M, Verouden NJ, et al. Genous endothelial progenitor cell capturing stent vs the Taxus Liberte stent in patients with de novo coronary lesions with a high-risk of coronary restenosis: a randomized, single-centre, pilot study. Eur Heart J. 2010;31:1055–1064. | ||
Natsuaki M, Kozuma K, Morimoto T, et al. Final 3-year outcome of a randomized trial comparing second-generation drug-eluting stents using either biodegradable polymer or durable polymer: NOBORI biolimus-eluting versus XIENCE/PROMUS everolimus-eluting stent trial. Circ Cardiovasc Interv. 2015;8:e002817. | ||
Urban P, Meredith IT, Abizaid A, et al. Polymer-free drug-coated coronary stents in patients at high bleeding risk. N Engl J Med. 2015;373:2038–2047. | ||
Dudek D, Onuma Y, Ormiston JA, Thuesen L, Miquel-Hébert K, Serruys PW. Four-year clinical follow-up of the ABSORB everolimus-eluting bioresorbable vascular scaffold in patients with de novo coronary artery disease: the ABSORB trial. Eurointervention. 2012;7:1060–1061. | ||
Ormiston JA, Serruys PW, Onuma Y, et al. First serial assessment at 6 months and 2 years of the second generation of ABSORB everolimus-eluting bioresorbable vascular scaffold: a multi-imaging modality study. Circ Cardiovasc Interv. 2012;5:620–632. | ||
Serruys PW, Chevalier B, Dudek D, et al. A bioresorbable everolimus-eluting scaffold versus a metallic everolimus-eluting stent for ischaemic heart disease caused by de-novo native coronary artery lesions (ABSORB II): an interim 1-year analysis of clinical and procedural secondary outcomes from a randomised controlled trial. Lancet. 2015;385:43–54. | ||
Cutlip DE, Windecker S, Mehran R. Clinical end points in coronary stent trials: a case for standardized definitions. Circulation. 2007; 115:2344–2351. | ||
Cutlip DE, Baim DS, Ho KK. Stent thrombosis in the modern era: a pooled analysis of multicenter coronary stent clinical trials. Circulation. 2001;103:1967–1971. | ||
Iakovou I, Schmidt T, Bonizzoni E. Incidence, predictors, and outcome of thrombosis after successful implantation of drug-eluting stents. JAMA. 2005;293:2126–2130. | ||
Wenaweser P, Rey C, Eberli FR. Stent thrombosis following bare-metal stent implantation: success of emergency percutaneous coronary intervention and predictors of adverse outcome. Eur Heart J. 2005;26:1180–1187. | ||
Stone GW, Moses JW, Ellis SG. Safety and efficacy of sirolimus- and paclitaxel-eluting coronary stents. N Engl J Med. 2007;356:998–1008. | ||
Eisenstein EL, Anstrom KJ, Kong DF. Clopidogrel use and long-term clinical outcomes after drug-eluting stent implantation. JAMA. 2007;297:159–168. | ||
Kastrati A, Mehilli J, Pache J, et al. Analysis of 14 trials comparing sirolimus-eluting stents with bare-metal stents. N Engl J Med. 2007; 356:1030–1039. | ||
Popma JJ, Weiner B, Cowley MJ, Simonton C, McCormick D, Feldman T. FDA advisory panel on the safety and efficacy of drug-eluting stents: summary of findings and recommendations. J Interv Cardiol. 2007;20:425–446. | ||
Baber U, Mehran R, Sharma SK, et al. Impact of the everolimus-eluting stent on stent-thrombosis: a meta-analysis of 13 randomized trials. J Am Coll Cardiol. 2011;58:1569–1577. | ||
Palmerini T, Kirtane AJ, Serruys PW, et al. Stent thrombosis with everolimus-eluting stents: meta-analysis of comparative randomized controlled trials. Circ Cardiovasc Interv. 2012;5:357–364. | ||
Raber L, Magro M, Stefanini GG, et al. Very late coronary stent thrombosis of a newer-generation everolimus-eluting stent compared with early-generation drug-eluting stents: a prospective cohort study. Circulation. 2012;125:1110–1121. | ||
von Birgelen C, Basalus MW, Tandjung K, et al. A randomized controlled trial in second-generation zotarolimus-eluting Resolute stents versus everolimus-eluting Xience V stents in real-world patients: the TWENTE trial. J Am Coll Cardiol. 2012;59:1350–1361. | ||
Palmerini T, Biondi-Zoccai G, Della Riva D, et al. Stent thrombosis with drug-eluting and bare-metal stents: evidence from a comprehensive network meta-analysis. Lancet. 2012;379:1393–1402. | ||
Nakano M, Yahagi K, Otsuka F, et al. Causes of early stent thrombosis in patients presenting with acute coronary syndrome: an ex vivo human autopsy study. J Am Coll Cardiol. 2014;63:2510–2520. | ||
Van Werkum JW, Heestermans AA, Zomer AC, et al. Predictors of coronary stent thrombosis: the Dutch Stent Thrombosis Registry. J Am Coll Cardiol. 2009;53:1399–1409. | ||
Mauri L, Hsieh WH, Massaro JM, Ho KK, D’Agostino R, Cutlip DE. Stent thrombosis in randomized clinical trials of drug-eluting stents. N Engl J Med. 2007;356:1020–1029. | ||
Kuchulakanti PK, Chu WW, Torguson R, et al. Correlates and long-term outcomes of angiographically proven stent thrombosis with sirolimus- and paclitaxel-eluting stents. Circulation. 2006;113:1108–1113. | ||
Ge L, Airoldi F, Iakovou I, et al. Clinical and angiographic outcome after implantation of drug-eluting stents in bifurcation lesions with the crush stent technique: importance of final kissing balloon post-dilation. J Am Coll Cardiol. 2005;46:613–620. | ||
Park SJ, Kang SJ, Virmani R, Nakano M, Ueda Y. In-stent neoatherosclerosis. A final common pathway of late stent failure. J Am Coll Cardiol. 2012;59:2051–2057. | ||
Joner M, Finn AV, Farb A. Pathology of drug-eluting stents in humans: delayed healing and late thrombotic risk. J Am Coll Cardiol. 2006; 48:193–202. | ||
Virmani R, Guagliumi G, Farb A. Localized hypersensitivity and late coronary thrombosis secondary to a sirolimus-eluting stent: should we be cautious? Circulation. 2004;109:701–705. | ||
Tsimikas S. Drug-eluting stents and late adverse clinical outcomes: lessons learned, lessons awaited. J Am Coll Cardiol. 2006;47:2112–2115. | ||
Tanabe K, Serruys PW, Degertekin M. Incomplete stent apposition after implantation of paclitaxel-eluting stents or bare metal stents: insights from the randomized TAXUS II trial. Circulation. 2005;111:900–905. | ||
Chin-Quee SL, Hsu SH, Nguyen-Ehrenreich KL, et al. Endothelial cell recovery, acute thrombogenicity, and monocyte adhesion and activation on fluorinated copolymer and phosphorylcholine polymer stent coatings. Biomaterials. 2010;31:648–657. | ||
Kim MS, Dean LS. In-stent restenosis. Cardiovasc Ther. 2011;29:190–198. | ||
Kastrati A, Schomig A, Elezi S, et al. Predictive factors of restenosis after coronary stent placement. J Am Coll Cardiol. 1997;30:1428–1436. | ||
Komatsu R, Ueda M, Naruko T, Kojima A, Becker AE. Neointimal tissue response at sites of coronary stenting in humans: macroscopic, histological, and immunohistochemical analyses. Circulation. 1998;98:224–233. | ||
Kastrati A, Dibra A, Mehilli J, et al. Predictive factors of restenosis after coronary implantation of sirolimus- or paclitaxel-eluting stents. Circulation. 2006;113:2293–2300. | ||
Barlis P, Regar E, Serruys PW, et al. An optical coherence tomography study of a biodegradable vs. durable polymer-coated limus-eluting stent: a LEADERS trial sub-study. Eur Heart J. 2010;31:139–142. | ||
Nakazawa G, Finn AV, Vorpahl M, Ladich ER, Kolodgie FD, Virmani R. Coronary responses and differential mechanisms of late stent thrombosis attributed to first-generation sirolimus- and paclitaxel-eluting stents. J Am Coll Cardiol. 2011;57:390–398. | ||
Hamburger SA, McEver RP. Gmp-140 mediates adhesion of stimulated platelets to neutrophils. Blood. 1990;75:550–554. | ||
McEver RP, Cummings RD. Role of PSGL-1 binding to selectins in leukocyte recruitment. J Clin Invest. 1997;100:S97–S103. | ||
Caplice NM, Bunch TJ, Stalboerger PG, et al. Smooth muscle cells in human coronary atherosclerosis can originate from cells administered at marrow transplantation. Proc Natl Acad Sci U S A. 2003;100:4754–4759. | ||
Sata M, Saiura A, Kunisato A, et al. Hematopoietic stem cells differentiate into vascular cells that participate in the pathogenesis of atherosclerosis. Nat Med. 2002;8:403–409. | ||
Mathew V, Gersh BJ, Williams BA, et al. Outcomes in patients with diabetes mellitus undergoing percutaneous coronary intervention in the current era: a report from the Prevention of REStenosis with Tranilast and its Outcomes (PRESTO) trial. Circulation. 2004;109:476–480. | ||
Kobayashi Y, De Gregorio J, Kobayashi N, et al. Stented segment length as an independent predictor of restenosis. J Am Coll Cardiol. 1999;34:651–659. | ||
Hong MK, Park SW, Mintz GS, et al. Intravascular ultrasonic predictors of angiographic restenosis after long coronary stenting. Am J Cardiol. 2000;85:441–445. | ||
Farb A, Kolodgie FD, Hwang JY, et al. Extracellular matrix changes in stented human coronary arteries. Circulation. 2004;110:940–947. | ||
Kimura T, Abe K, Shizuta S, et al. Long-term clinical and angiographic follow-up after coronary stent placement in native coronary arteries. Circulation. 2002;105:2986–2991. | ||
Farb A, Heller PF, Shroff S, et al. Pathological analysis of local delivery of paclitaxel via a polymer-coated stent. Circulation. 2001; 104:473–479. | ||
Virmani R, Liistro F, Stankovic G, et al. Mechanism of late in-stent restenosis after implantation of a paclitaxel-derivate-eluting polymer stent system in humans. Circulation. 2002;106:2649–2651. | ||
Inoue K, Abe K, Ando K, et al. Pathological analyses of long-term intracoronary Palmaz–Schatz stenting: is its efficacy permanent? Cardiovasc Pathol. 2004;13:109–115. | ||
Otsuka F, Vorpahl M, Nakano M, et al. Pathology of second-generation everolimus-eluting stents versus first-generation sirolimus- and paclitaxel-eluting stents in humans. Circulation. 2014;129:211–223. | ||
Weidinger FF, McLenachan JM, Cybulsky MI, et al. Persistent dysfunction of regenerated endothelium after balloon angioplasty of rabbit iliac artery. Circulation. 1990;81:1667–1679. | ||
Joner M, Nakazawa G, Finn AV, et al. Endothelial cell recovery between comparator polymer-based drug-eluting stents. J Am Coll Cardiol. 2008;52:333–342. | ||
Lee CW, Kang SJ, Park DW, et al. Intravascular ultrasound findings in patients with very late stent thrombosis after either drug-eluting or bare-metal stent implantation. J Am Coll Cardiol. 2010;55:1936–1942. | ||
McDaniel MC, Eshtehardi P, Sawaya FJ, Douglas JS, Samady H. Contemporary clinical applications of coronary intravascular ultrasound. J Am Coll Cardiol Intv. 2011;4:1155–1167. | ||
Nair A, Kuban BD, Tuzcu EM, Schoenhagen P, Nissen SE, Vince DG. Coronary plaque classification with intravascular radiofrequency data analysis. Circulation. 2002;106:2200–2206. | ||
Kang SJ, Mintz GS, Park DW, et al. Tissue characterization of in-stent neointima using intravascular ultrasound radiofrequency data analysis. Am J Cardiol. 2010;106:1561–1565. | ||
Yamaguchi T, Terashima M, Akasaka T, et al. Safety and feasibility of an intravascular optical coherence tomography image wire system in the clinical setting. Am J Cardiol. 2008;101:562–567. | ||
Tearney GJ, Waxman S, Shishkov M, et al. Three-dimensional coronary artery microscopy by intracoronary optical frequency domain imaging. J Am Coll Cardiol Img. 2008;1:752– 61. | ||
Lee SY, Shin DH, Mintz G, et al. Optical coherence tomography-based evaluation of in-stent neoatherosclerosis in lesions with more than 50% neointimal cross-sectional area stenosis. Eurointervention. 2013;9:945–951. | ||
Kawamori H, Shite J, Shinke T, et al. The ability of optical coherence tomography to monitor percutaneous coronary intervention: detailed comparison with intravascular ultrasound. J Invasive Cardiol. 2010;22:54–55. | ||
Habara M, Terashima M, Suzuki T. Detection of atherosclerotic progression with rupture of degenerated in-stent intima five years after bare-metal stent implantation using optical coherence tomography. J Invasive Cardiol. 2009;21:552–553. | ||
Takano M, Yamamoto M, Inami S, et al. Appearance of lipid-laden intima and neovascularization after implantation of bare-metal stents extended late-phase observation by intracoronary optical coherence tomography. J Am Coll Cardiol. 2009;55:26–32. | ||
Kang SJ, Mintz GS, Akasaka T, et al. Optical coherent tomographic analysis of in-stent neo-atherosclerosis after drug-eluting stent implantation. Circulation. 2011;123:2913–2915. |
© 2017 The Author(s). This work is published and licensed by Dove Medical Press Limited. The
full terms of this license are available at https://www.dovepress.com/terms.php
and incorporate the Creative Commons Attribution
- Non Commercial (unported, v3.0) License.
By accessing the work you hereby accept the Terms. Non-commercial uses of the work are permitted
without any further permission from Dove Medical Press Limited, provided the work is properly
attributed. For permission for commercial use of this work, please see paragraphs 4.2 and 5 of our Terms.