Back to Journals » Drug Design, Development and Therapy » Volume 12

Chitosan–alginate BSA-gel-capsules for local chemotherapy against drug-resistant breast cancer
Authors Shen H, Li F, Wang D, Yang Z, Yao C, Ye Y, Wang X
Received 24 November 2017
Accepted for publication 16 February 2018
Published 19 April 2018 Volume 2018:12 Pages 921—934
DOI https://doi.org/10.2147/DDDT.S158001
Checked for plagiarism Yes
Review by Single anonymous peer review
Peer reviewer comments 2
Editor who approved publication: Dr Georgios Panos
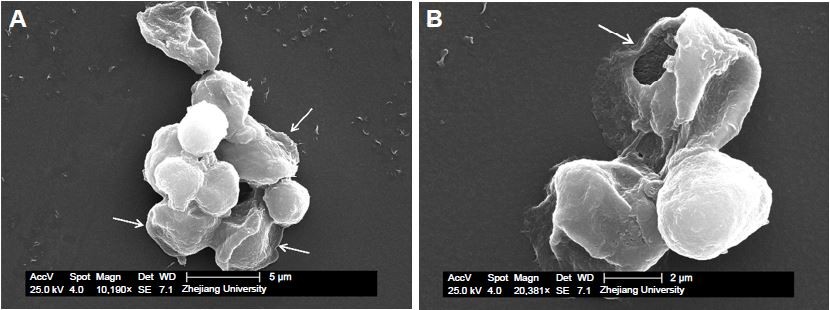
Haijun Shen,1 Fang Li,1 Dongxia Wang,1 Zhihan Yang,1 Chunfang Yao,1 Yang Ye,1 Xiaona Wang2
1Department of Preventive Medicine and Public Health Laboratory Science, School of Medicine, Jiangsu University, Zhenjiang, Jiangsu Province, China; 2Department of Internal Medicine of Jiangsu University Hospital Workers, The Affiliated Hospital of Jiangsu University, Zhenjiang, Jiangsu Province, China
Background and object: Polyelectrolyte microcapsule is a promising candidate for multifunctional drug delivery system. However, the lack of reports about animal experiments have greatly slowed down their development for drug delivery. We engineered biodegradable chitosan–alginate polyelectrolyte multilayer capsule filled with bovine serum albumin gel (BSA-gel-capsule). Herein, we demonstrated their applicability for local chemotherapy, a means of treating local or regional malignancies by direct administration of anti-tumor agents to tumor sites.
Method: Doxorubicin (DOX) was loaded in BSA-gel-capsules and DOX-resistant cell line (MCF-7/ADR cells) was employed for antitumor studies in vitro. The cytotoxicity, cellular uptake and distribution of DOX from BSA-gel-capsules were studied. Afterwards, MCF-7/ADR xenografts tumor model was established in nude mice. The in vivo antitumor efficacy of DOX-loaded BSA-gel-capsules by intratumor injection was then evaluated.
Result: Compared with free DOX, more effective cytotoxicity against MCF-7/ADR cells after treatment with DOX-loaded BSA-gel-capsules was revealed, demonstrating the positive reversal effect on drug-resistance. Thereafter, the more cellular uptake and nucleus distribution of DOX from BSA-gel-capsules in MCF-7/ADR cells provided convincing explanation for the reversal effect. DOX-loaded BSA-gel-capsules displayed remarkably more antitumor efficacy than free DOX in MCF-7/ADR cell-xenografted mice. Finally, the high DOX accumulation and prolonged retention in tumor site after local administration of DOX-loaded BSA-gel-capsules was demonstrated, displaying the unique advantages of BSA-gel-capsules for local chemotherapy.
Conclusion: These findings indicate that DOX-loaded BSA-gel-capsules should be considered a potential candidate for the treatment of drug-resistant breast cancer. This paper provides a feasibility for the local chemotherapy of polyelectrolyte microcapsules, which will be a big step towards their application as drug delivery vehicles.
Keywords: polyelectrolyte capsule, bovine serum albumin, layer-by-layer, pH-responsive, intratumor injection, multidrug resistance
Introduction
Polyelectrolyte multilayer capsules, assembled using the layer-by-layer (LbL) technique, have attracted much attention in drug delivery due to their ease of preparation, the multifunctionality, and responsiveness to various stimuli.1–4 Over the last decades, efforts have been devoted to loading various substances into preformed hollow capsules.5–8 However, the loading amount of desired substances, especially for drugs, is insufficient to carry out a large-scale evaluation, such as animal experiments.9 More recently, spontaneous deposition emerged as a promising method for encapsulation.10–12 It occurs in the case of electrostatic attraction between the charged molecules and an oppositely charged matrix inside the capsules, thus facilitating accumulation of molecules into the preformed capsules. The accumulation of drugs in the capsules can achieve ten to hundred times of the feeding drug concentration. This method possesses the virtues of high encapsulation efficiency and good reproducibility but also has the disadvantage of inefficient release, because the encapsulated molecules are bound with the matrix tightly driven by electrostatic attraction. Focused on the strategy for overcoming this disadvantage, in our previous study, we have engineered a novel polyelectrolyte microcapsule packaging BSA (bovine serum albumin) gels, termed BSA-gel-capsule. These capsules not only showed pronounced spontaneous encapsulation of doxorubicin (DOX) into capsules, but also displayed excellent pH-sensitive release, owing to the charge variability of BSA with changes in pH.13,14 The schematic diagram of BSA-gel-capsule and its spontaneous loading at pH 6.5 and sustained release at pH 5.0 are depicted in Figure 1.
Polyelectrolyte multilayer capsules have been used in many applications in addition to usage in fundamental science. Applications in medical fields such as cancer treatment have been paid special attention.15 Although many anticancer drugs have been loaded in polyelectrolyte microcapsules and their in vitro cell viability/cytotoxicity has been extensively reported, the in vivo antitumor studies at the level of animal experimentation are very little. Unfortunately, animal experiments are absolutely necessary for progress toward application for drug delivery. Therefore, in our previous study, we fabricated BSA-gel-capsules with an average diameter of 5.4±0.9 μm and evaluated the feasibility of using BSA-gel-capsules in vivo by intravenous (IV) injection. A remarkable targeting action to the lung and effective inhibition of the pulmonary melanoma growth were discovered after IV injection of DOX-loaded BSA-gel-capsules. The results demonstrated the applicability of polyelectrolyte microcapsules as drug delivery by IV injection for the first time.13,14
Herein, in order to broaden the application of BSA-gel-capsules and even polyelectrolyte microcapsules in the drug delivery system, we intend to apply BSA-gel-capsules as a novel drug delivery vehicle for local chemotherapy. As we all know, the chemotherapy effect of cancer treatment mainly depends on the accumulated concentrations and persistent periods of drugs in tumor site. Local chemotherapy, a kind of interventional therapy method, provides a means of treating local or regional malignancies by direct administration of antitumor agents to tumor sites. This method exhibits unique advantages in increasing drug concentrations because drugs are injected or implanted directly into tumor site.16 Unfortunately, different from the conventional injection with syringe, injection and implantation for local chemotherapy must need the help of interventional devices in clinics, such as the endoscopic ultrasound-guided fine needle injection. Therefore, the frequency of drug administration should be better as little as possible. Considering the sustained release behavior of DOX-loaded BSA-gel-capsules in the tumor acidic microenvironment, we speculate that BSA-gel-capsules will be optimal for local chemotherapy. Employment of DOX-loaded BSA-gel-capsules will greatly increase DOX concentration and retention time in cancerous tissue. These virtues may translate into minimized systemic side effects, reduced doses, and less frequent administration, which are much beneficial to local chemotherapy (Figure 1).
For this context, on the basis of our previous study, DOX-loaded BSA-gel-capsules were constructed using chitosan and alginate as the capsule shell components. DOX release behavior from BSA-gel-capsules at pH 5.0 was depicted. Afterward, to evaluate the applicability of BSA-gel-capsules for local chemotherapy, in vitro cytotoxicity (to MCF-7 cells and DOX-resistant cell line MCF-7/ADR cells) and in vivo antitumor efficacy (in an MCF-7/ADR xenografts tumor model) of DOX-loaded BSA-gel-capsules were further studied. In particular, for the first time, we examined the reversal effect of DOX-loaded BSA-gel-capsules on multidrug resistance of cancer cells. This study is envisaged to facilitate the development of biomedical applications of polyelectrolyte microcapsules, especially for drug delivery and cancer treatment.
Materials and methods
Materials
Chitosan was provided by Aoxing Biotechnology Co. Ltd. (Zhejiang, China). Sodium alginate and MTT were purchased from Sigma-Aldrich. BSA (fraction V, 99%) was supplied by Aoduofuni Biotechnology Co. Ltd. (Nanjing, China). Doxorubicin hydrochloride was from Haikou Manfangyuan Chemical Co., Ltd. (Haikou, China). LysoTracker Green and Hoechst 33342 were from Beyotime Institute of Biotechnology (Jiangsu, China). All other reagents, which were of analytical grade, were obtained from Sinopharm Chemical reagent Co., Ltd. (Shanghai, China). All solutions were prepared by ultrapure water which was filtered by 0.22-μm pore size filter equipped in a Milli-Q system (Millipore Corporation, Billerica, MA, USA).
Cell lines and animals
The human breast cancer cell lines (MCF-7 cells and DOX-resistant cell line MCF-7/ADR cells) were kindly provided by the Cancer Institute of the Second Affiliate Hospital of Zhejiang University School of Medicine. They purchased MCF-7 cell line from the Chinese Academy of Sciences Cell Bank of Type Culture Collection (Shanghai, China). MCF-7 cells were cultured in Roswell Park Memorial Institute (RPMI) 1640 medium supplemented with 10% (v/v) FBS and antibiotics (100 U/mL penicillin and streptomycin). MCF-7/ADR cells were grown in complete RPMI 1640 medium with 1 μg/mL DOX. Both cell lines were maintained at 37°C in a humidified 5% CO2 atmosphere. MCF-7/ADR cells were cultured in complete RPMI 1640 medium (no DOX) 1 week before experiment. Female BALB/c nude mice of 4 weeks were purchased from Slac Laboratory Animal Co., Ltd. (Shanghai, China) and kept under specific pathogen-free conditions. All animal experiments were in accordance with the guidelines approved by the Institutional Animal Care and Use Committee of Zhejiang University for the welfare of the experimental animals, and all protocols were performed with the approval of the Ethical Committee of Zhejiang University (China).
Fabrication of BSA-gel-capsules
BSA-gel-capsules were fabricated as follows. In the first step, CaCO3 microparticles doped with BSA molecules, abbreviated as CaCO3 (BSA), were prepared by pouring Na2CO3 solution into BSA and CaCl2 solution rapidly. After intense agitation for 30 s, CaCO3 (BSA) particles were formed and collected by centrifugation and dried in vacuum. In the second step, five (chitosan/alginate) bilayers were alternatively deposited on CaCO3 (BSA) particles using LbL technology. These core–shell particles were cross-linked with 2% glutaraldehyde at room temperature for 12 h. Thereafter, they were shaken in 0.2 M EDTA solution four times, assuring the complete dissolution of the CaCO3 templates. The obtained capsules were washed and redispersed with deionized water. Then, they were heated at 80°C for 1 h under gentle agitation to induce the gelation of BSA in the capsules. The suspension of the resultant chitosan–alginate capsules filled with BSA gels, BSA-gel-capsules in short, was obtained and kept at 4°C before use. Herein, the zeta potentials of chitosan solution and alginate solution used for LbL deposition were monitored by Malvern Zetasizer 3000HS (Malvern Instruments, Malvern, UK). Moreover, we obtained the diameter of the BSA-gel-capsules with the help of confocal laser scanning microscope (CLSM).9
Drug encapsulation and in vitro release
As reported previously, (2.0±0.3) ×107 BSA-gel-capsules were mixed with 500 μL of 1,000 μg/mL DOX solution at pH 6.5. After incubation for 12 h at room temperature, the mixture was centrifuged at 4,000 rpm for 5 min and thus free DOX was separated in supernatant solution. After diluting 200 μL of the supernatant to 10 mL with deionized water, the absorbance at 253 nm was measured by a UV–visible spectrophotometer (TU-1800PC; Persee, Beijing, China). Raw data were converted to the original supernatant concentration by referring to a calibration curve. The encapsulation efficiency of DOX and the concentration of DOX loaded in BSA-gel-capsules (Cc) were thus calculated by the following equations:
Here Vc represents the total volume of BSA-gel-capsules used for DOX loading. The average diameter of BSA-gel-capsules is 5.4 μm (assessed by averaging 100 capsules under CLSM), so the average capsule volume is calculated as 8.2×10−11 mL. The number of BSA-gel-capsules was counted using a hemocytometer under a microscope.
The DOX accumulation in BSA-gel-capsules was further qualitatively described by means of CLSM (LSM 510 META; ZEISS, Oberkochen, Germany). A droplet of the DOX-loaded capsules suspension was dripped onto a glass slide, covered with a coverslip and then observed. The confocal images and DIC (differential interference contrast) images of DOX-loaded BSA-gel-capsules were then obtained.
The DOX release behavior from BSA-gel-capsules at pH 5.0 was studied. The DOX-loaded capsules were suspended in 1 mL PBS buffer at pH 5.0 and incubated at 37°C under gentle shaking. After immersed for a desired time, 900 μL of the supernatant was withdrawn by centrifugation and supplemented with 900 μL fresh PBS buffer. Each collected supernatant was diluted with PBS buffer at pH 5.0, the absorbance of DOX at 253 nm was recorded, and the cumulative release of DOX was calculated with the help of the standard curves (data not shown).
In vitro cytotoxicity assay
The in vitro cytotoxicity of DOX-loaded BSA-gel-capsules was assessed by MTT assay. Briefly, MCF-7 and MCF-7/ADR cells (5,000 cells/well) were seeded in 96-well plates and cultured overnight. Then the cells were exposed to serial concentrations of BSA-gel-capsules, free DOX, and DOX-loaded BSA-gel-capsules in culture medium, respectively (final DOX concentration of 0.05, 0.2, 1, 5, 20, 50 μg/mL). As controls, cells were incubated with normal culture medium. After cultivation for 24 h, the medium was removed, and the cells were washed carefully with PBS and incubated with fresh medium containing 0.5 mg/mL MTT for another 4 h. Medium was removed again, and DMSO (200 μL/well) was added. The plates were incubated at 37°C for 10 min, and then the absorbance was recorded at 570 nm using a microplate reader (Bio-Rad, Hercules, CA, USA).
Cellular uptake of DOXs
The cellular uptake and intracellular distribution of DOX were evaluated using CLSM (LSM 510 META). MCF-7 cells and MCF-7/ADR cells were inoculated into 6-well plates with densities of 2×105 cells per well and cultured overnight. The cells were then treated with either free DOX or DOX-loaded BSA-gel-capsules (DOX concentration of 1 μg/mL). After incubation for another 24 h, the medium was discarded and the cells were carefully washed with PBS. Afterward, the lysosomes of the cells were stained by LysoTracker Green for 30 min. At last, cells were washed with PBS for three times and fixed with 4% (w/v) paraformaldehyde. The intracellular localization of DOX was observed with CLSM.
For quantitative analysis of the cellular uptake, MCF-7 cells and MCF-7/ADR cells were cultured in 6-well plates and treated with either free DOX or DOX-loaded BSA-gel-capsules as described above. At time points of 1, 4, and 24 h post treatment, cells were washed with PBS, trypsinized, and centrifuged at 1,000 rpm for 5 min to obtain a cell pellet, which was subsequently resuspended in PBS and analyzed using a flow cytometer (BD Biosciences, San Jose, CA, USA).
In vivo antitumor activity
For the breast cancer model, three nude mice bearing MCF-7/ADR xenografts were first generated by injection of 5×106 MCF-7/ADR cells into each mouse at the right axilla. When the tumors reached 0.5×0.5 cm in diameter, they were harvested, cut into uniform pieces, and implanted into the right axilla of other nude mice. The implanted tumors were allowed to grow to ~0.5×0.5 cm in diameter. Then the mice were divided randomly into three groups: control (100 μL physiological saline), free DOX, and DOX-loaded BSA-gel-capsules. DOX (2 mg/kg mouse weight) and equivalent dose of DOX-loaded BSA-gel-capsules were injected directly into the tumor every 4 days. After injection for five times, the mice were sacrificed, and their tumors were excised, weighed, photographed, and quickly fixed with formalin for further histopathological examination. After immersion in formalin for at least 24 h, the tumors were embedded in paraffin, sectioned, stained with H&E, and examined under a light microscope. Histopathologies between groups were compared. The tumor inhibitory rate (TIR) was calculated using the following equation:
where Wtest is the mean tumor weight of the tested groups and Wsaline refers to the mean tumor weight of the saline group.
Biodistribution examination of DOX
In order to study the biodistribution of DOX from DOX-loaded BSA-gel-capsules, we employed the Maestro in vivo imaging system (CRi, Shawano, WI, USA). Briefly, the mice bearing MCF-7/ADR xenografts were administered with free DOX or DOX-loaded BSA-gel-capsules using a DOX dose of 2 mg/kg via intratumor injection. At time points of 1 and 4 days post injection, mice were sacrificed and the tumor and major organs were harvested. Each organ was rinsed with PBS three times and put into the board, and the fluorescent images were detected.
Statistical analysis
Statistical analysis of the tumor weight after different treatments was performed using one-way analysis of variance (ANOVA) with Tukey post-hoc test. Differences between groups were considered statistically significant at P<0.05 (*). The data distribution was tested in SPSS Statistics 23.0 (IBM Corporation, Armonk, NY, USA) using Shapiro–Wilk test of normality. The power analysis was performed with PASS 11 software (NCSS, LLC, Kaysville, UT, USA), and the alpha (significant level) value was set at 0.05.
Results
Fabrication of BSA-gel-capsules
BSA-gel-capsules were obtained by a two-step process, as described in our previous work.14 First, CaCO3 (BSA) microparticles were obtained through mineralization of the solutions of Na2CO3 and CaCl2 in the presence of BSA. According to this method, BSA can be integrated into growing CaCO3 particles and practically cannot be released without dissolving the matrix. Then, five bilayers of (chitosan/alginate) were assembled on CaCO3 (BSA) microparticles using the LbL assembly method. The zeta potentials of chitosan solution and alginate solution were 22.5±6.2 mV and −17.3±5.0 mV, respectively. The opposite charges ensured the alternative deposition of chitosan and alginate on templates by electrostatic attractions. After crosslinking by glutaraldehyde, the five bilayers formed a stable shell around CaCO3 template. Thereafter, abundant BSA molecules were kept inside each capsule after dissolution of CaCO3 template by EDTA. By heat treatment at 80°C for 1 h, denaturation and gelation of BSA inside the capsule proceeded in a sequential process, and finally BSA-gel-capsule was obtained. BSA-gel-capsules with alginate as the outermost layer were negatively charged, and the zeta potential was −20.4±5.5 mV.
Herein, chitosan and alginate were employed as the capsule shell components, because both of them are polysaccharides with good biocompatibility. For biomedical applications, such as drug delivery, the components should be biodegradable and biocompatible to avoid the occurrence of side effects. Recently, there has been an increasing interest in fabricating capsules with polypeptides and polysaccharides.9
SEM observation (Figure 2A) showed that the capsules were spherical and rigid enough to stand like microparticles, probably due to the solidification by glutaraldehyde and the swelling of BSA gels. However, it is still visible that particulate-gels were packaged in BSA-gel-capsules by thin shells, indicated by arrows in Figure 2A. This was further confirmed by Figure 2B; the image obviously shows that there was a thin shell and the capsule was collapsed when BSA gels leaked out from the broken wall.
Drug encapsulation and in vitro release
According to our previous work, BSA-gel-capsules showed the remarkable variation of the DOX loading along with pH changes (pH 2.0~6.5). In this text, DOX was loaded into BSA-gel-capsules by stirring at pH 6.5. BSA is a polyampholyte and its isoelectric point (pI) is 4.8.17 Thus, BSA gels inside the microcapsules have net negative charges above their pI (pH 6.5), and the driving force for DOX loading was generated by electrostatic attraction between BSA and positively charged DOX (Figure 1). Herein, DOX concentrations in capsules (115.7 mg/mL) were >100 times higher than in supernatant (0.62 mg/mL), demonstrating a pronounced DOX accumulation into the capsules. This effect has previously been recognized as spontaneous deposition driven by electrostatic attraction between positively charged drugs and negatively charged polymers in capsules. The DOX encapsulation efficiency reached 38.0%±2.0%, indicating BSA-gel-capsule was indeed an effective DOX-loading vehicle.
The DOX loading was further confirmed by CLSM. The DIC image (Figure 3B) showed that there were dark and nontransparent objects in BSA-gel-capsules. This is consistent with our previous report.14 The dark and nontransparent objects had been recognized as BSA gels in the interiors of capsules. Strong fluorescence emission from DOX molecules was recorded from the interiors of BSA-gel-capsules and overlapped with the BSA gels (Figure 3A and C), indicating that DOX was encapsulated into BSA-gel-capsules by electrostatic attraction between the positively charged DOX molecules and the negatively charged BSA gels.
The DOX release behavior from BSA-gel-capsules at pH 5.0 was depicted, because the tumor microenvironment has an acidic pH of ca. 5.0. As shown in Figure 4, the release was rapid at the initial stage, after which it became sustained. This can be explained by the electrostatic interaction (between BSA and DOX) and the large concentration gradient (between the capsule interior and the bulk). At pH 5.0, the electrostatic interaction was negligible, and the large concentration gradient resulted in a rapid release at the initial stage. After that, the concentration gradient was narrowed and the release became sustained consequently. DOX release curves at the initial stage (between 0 and 120 min) at pH 5.0 were fit by equations. The best fit with highest correlation (r=0.9608) was observed with Higuchi’s model compared with zero-order model and first-order model, indicating that the release mechanism of the DOX-loaded BSA-gel-capsules was primarily diffusion controlled. It is reasonable considering the intrinsic permeability of polyelectrolyte microcapsule walls. Molecules can be loaded into or released from capsules by diffusion through the permeable walls, as described in many previous reports.1,18
In vitro cytotoxicity assay
The in vitro cytotoxicity of blank BSA-gel-capsules was evaluated on MCF-7 and MCF-7/ADR cells. MTT assay was used to evaluate the cytotoxicity. As shown in Figure 5A and B, the viability was not significantly affected at concentrations up to 2.5×106 capsules, indicating that BSA-gel-capsule has almost no cytotoxicity and has a good biocompatibility. The negligible viability decrease at high capsule concentration is considered to be caused by aggregation of capsules on the bottom of 96-well plates, which compressed the space for cell growth.15,19
Then, the cytotoxicity of DOX-loaded BSA-gel-capsules was further investigated. For MCF-7 cells (Figure 5C), both free DOX and encapsulated DOX exhibited dose-dependent cytotoxicity, indicating that DOX-loaded BSA-gel-capsules were also able to inhibit cell growth and play the antitumor effect on MCF-7 cells. For MCF-7/ADR cells (Figure 5D), free DOX showed little cytotoxicity even at DOX concentrations up to 20 μg/mL. In contrast, the cytotoxicity of DOX-loaded BSA-gel-capsules against MCF-7/ADR cells was significantly higher, demonstrating the reversal effect of DOX-loaded BSA-gel-capsules on multidrug resistance of MCF-7/ADR cells. This result is very interesting because there are little reports about the drug-resistance reversal effect of microcapsules, although this effect of nanoparticles has been widely studied.
Cellular uptake of DOX
For further study and confirmation of the multidrug-resistance reversal effect generated by DOX-loaded BSA-gel-capsules, the cellular uptake of DOX in MCF-7 and MCF-7/ADR cells was evaluated. The DOX-positive cells were quantitatively assessed by flow cytometry after being incubated for 1, 4, and 24 h. As shown in Figure 6A, in MCF-7 cells, the cellular uptake of free DOX and DOX from capsules was time dependent and almost saturated to 100% at 4 h. However, as shown in Figure 6B, the uptake of free DOX in MCF-7/ADR cells was low, while the uptake of DOX from capsules was much higher. The amount of positive cells, after incubation with DOX-loaded BSA-gel-capsules for 24 h, was approximately tenfold that of free DOX in MCF-7/ADR cells.
Afterward, in order to observe the intracellular distribution of DOX, LysoTracker Green was used to stain the lysosomes. Figure 7 shows the location of DOX in MCF-7 and MCF-7/ADR cells. In MCF-7 cells, after incubation with DOX-loaded capsules for 24 h, cells showed strong red fluorescence in nucleus, which was the same as cells treated with free DOX, indicating that DOX could be released from BSA-gel-capsules and enter the nucleus to play the cytotoxic effect. While in MCF-7/ADR cells, only a small amount of free DOX was distributed in the cytoplasm and could not enter the nucleus. Interestingly, when MCF-7/ADR cells were treated with DOX-loaded capsules, the red fluorescence of intracellular DOX was significantly increased. The result is consistent with the above cellular uptake. Additionally, the red fluorescence was distributed in both cytoplasm and nucleus, suggesting that the chemotherapy drug could be transported to cell nucleus with the help of BSA-gel-capsules. These results provide intuitional proof and explanation for the multidrug-resistance reversal effect generated by BSA-gel-capsules.
Previous reports have identified the apoptosis as the predominant mechanism of cell death in response to DOX treatment.20,21 If DOX-loaded vectors (eg, nanoparticles and microparticles) are able to be taken up to further release DOX in cancer cells, the apoptosis will be induced. In our previous report, we had demonstrated the cell apoptosis of the murine melanoma B16F10 cells induced by DOX-loaded BSA-gel-capsules.14 The chromatin condensation and nuclei fragmentation, which are signs of cell apoptosis,22,23 were clearly observed in B16F10 cells. However, in multidrug-resistance cells, DOX can be excluded out of the cells by drug efflux pumps (especially P-glycoprotein, P-gp). Therefore, the cytotoxicity of MCF-7 and MCF-7/ADR cells mainly depends on whether DOX can be taken up and distributed in cells. In this study, the favorable cellular uptake of DOX from BSA-gel-capsules was confirmed by flow cytometry and the nucleus distribution was exhibited by CLSM. Hence, the cytotoxicity of MCF-7 and MCF-7/ADR cells induced by DOX-loaded BSA-gel-capsules was credible and reasonable.
In vivo antitumor studies
To evaluate the antitumor efficacy of DOX-loaded BSA-gel-capsules in vivo, we established the tumor model of nude mice bearing MCF-7/ADR tumors. After treatment in protocol, mice were sacrificed, and their tumors were excised, photographed, and weighed. The different antitumor activities are depicted in Figure 8. The tumor-bearing mice after treatment for 24 days were shown in Figure 8A, while the excised tumors were shown in Figure 8B. The mice treated with saline had a large tumor volume, whereas the mice treated with DOX-loaded capsules displayed significantly reduced tumor size. The tumor weight after different treatments was then analyzed using one-way ANOVA. First, the normal distribution of data, which is necessary for ANOVA, was tested (Table S1). The significance values were all >0.05, demonstrating the normality. Thereafter, one-way ANOVA was conducted to analyze the differences between groups (raw result is shown in Table S2). Compared with saline treatment, as depicted in Figure 9, free DOX treatment significantly reduced the tumor weight (P=0.002). Moreover, DOX-loaded capsules treatment showed significantly reduced tumor weight in comparison with free DOX treatment (P=0.006), indicating the better antitumor efficacy of DOX-loaded capsules. Power of all statistical tests was 0.99 (results and summary statements presented in Table S3). The inhibition rate of tumors, calculated with the tumor weights, is presented in Figure 9. DOX-loaded capsules showed the highest inhibition rate of 68.29%, while the free DOX showed only 37.07%. The H&E-stained tissue sections (Figure 10) show that there was a great deal of cancer cells after treatment with saline. These cancer cells had distinct nucleus and grew well (Figure 10A). After treatment with free DOX, some cell nucleus became fragmented (Figure 10B). In contrast, treatment with DOX-loaded capsules resulted in extensive necrosis of the tumor tissue (Figure 10C). These results demonstrate that both free DOX and DOX-loaded BSA-gel-capsules could inhibit tumor growth. DOX-loaded BSA-gel-capsules were more effective, indicated by smaller tumor size, higher inhibition rate, and larger necrotic region. Besides the drug-resistance reversal effect on MCF-7/ADR cells as mentioned in above sections, this can be well understood by the fact that DOX-loaded BSA-gel-capsules accumulated in tumors and played the therapeutic role for a long time.
Biodistribution examination of DOX
To support the above explanation of better tumor-inhibitory capacity of DOX-loaded BSA-gel-capsules, the prolonged retention of DOX-loaded capsules in tumors was observed using the in vivo imaging system. Figure 11 displays the fluorescence images of tumors and major organs at 1 and 4 days after intratumor injection of free DOX or DOX-loaded capsules. For DOX-loaded capsules, tumors at both days 1 and 4 showed strong fluorescence, indicating the high DOX accumulation and prolonged retention. For free DOX, however, the fluorescent intensity of tumor at day 4 was obviously weaker than the intensity at day 1, suggesting a faster metabolism and excretion. Therefore, DOX-loaded BSA-gel-capsules can accumulate and be retained in tumors, thus increasing the drug concentration and tumor-inhibitory period. In addition, as shown in Figure 11, there was little DOX distributed in organs (heart, liver, lung, spleen, and kidney), indicating that the systemic toxicity of DOX was negligible after intratumor injection of DOX-loaded BSA-gel-capsules. These results demonstrated the advantages of BSA-gel-capsules in the application of local chemotherapy.
Discussion
The low encapsulation efficiency of drugs and the lack of studies in vitro and in vivo have greatly slowed down the development of polyelectrolyte capsules for drug delivery, one of the envisioned core applications of these capsules. Herein, chitosan–alginate polyelectrolyte microcapsules packaging BSA gels, termed BSA-gel-capsules, were engineered. Owing to the charge variability of BSA with changing pH, these capsules not only can efficiently encapsulate DOX by spontaneous deposition at pH 6.5 but can also release DOX following a sustained behavior at pH 5.0. The effective loading and sustained release provided the foundation for application of BSA-gel-capsules in cancer treatment.
Polyelectrolyte microcapsules are expected to contribute to progress in cancer treatment.24,25 However, their large size (1–10 μm) and the targeted release of drugs to cancerous tissues remain the main challenges in their use as delivery vehicles in vivo. In this study, taking advantage of the sustained DOX release in the tumor acidic microenvironment, DOX-loaded BSA-gel-capsules were employed for local chemotherapy against breast cancer. Compared with free DOX, both DOX concentration and retention time in tumors were greatly increased by direct injection of DOX-loaded capsules to tumor sites. A more effective inhibition of xenografted tumors was also revealed.
In particular, we discovered the reversal effect of DOX-loaded capsules on multidrug resistance of cancer cells. Sustained release of antitumor agents increases tumor responsiveness and reduces the risk of multidrug resistance.16,26 In a previous report, DOX microspheres with a mean particle size of 4.9±1.2 μm were prepared, and their treatment of multidrug-resistant melanomas was studied. The results demonstrated that intracellular DOX concentrations were higher in drug-resistant tumor cells treated with microspheres as opposed to solution, and efflux of DOX from the tumor cell was inhibited. Greater cytotoxic effects were seen in tumor cells treated with DOX microspheres versus solution up to and after 3 days.26
Cancer cell is a kind of nonspecific phagocytic cell. Polyelectrolyte microcapsules can be internalized into cancer cells by lipid-raft-mediated uptake and then located at endosome/lysosome.27–29 As we all know, the endosome/lysosome has a low pH value about 4.5–5.0,18 which can facilitate the DOX release from BSA-gel-capsules as described in the Results section “Drug encapsulation and in vitro release”. That is, the high expression of P-gp in the drug-resistant cells would efflux free DOX, which entered the membrane through electrostatic interaction.30,31 However, DOX-loaded BSA-gel-capsules could enter the cells through lipid-raft-mediated uptake and bypass the drug efflux pumps, thus releasing DOX persistently and exhibiting the reversal effect of drug resistance.
Conclusion
We have described DOX-loaded BSA-gel-capsules with remarkable antitumor activities against drug-resistant breast cancer. Both free DOX and DOX-loaded BSA-gel-capsules effectively induced the apoptosis of MCF-7 cells. However, against MCF-7/ADR cells, the cytotoxicity of DOX-loaded BSA-gel-capsules was significantly higher than free DOX, demonstrating definite reversal effect on drug resistance. The more cellular uptake and nucleus distribution of DOX from BSA-gel-capsules in MCF-7/ADR cells provided reasonable explanation for the reversal effect. Afterward, the encapsulated DOX was applied to treat drug-resistant breast cancer in xenografted mice, revealing a longer retention time in tumor site and a better ability of tumor inhabitation than the same dosage of free DOX. Therefore, we highlight, for the first time, the applicability of BSA-gel-capsules for the treatment of drug-resistant breast cancer. Furthermore, the results also offered the applicability of the extensively studied polyelectrolyte microcapsules, especially in the fields of drug delivery for local chemotherapy.
Acknowledgments
This work was partially supported by the National Natural Science Foundation of China (Nos. 81402870 and 81502800), the Natural Science Foundation of Jiangsu Province for Youth (No. BK20140579), China Postdoctoral Science Foundation (Nos. 2015M571696, 2013M540424, and 2015T80513), the Jiangsu Province Postdoctoral Science Foundation (No. 1401145C), and the Scientific Research Foundation of Jiangsu University (No. 14JDG046).
Disclosure
The authors report no conflicts of interest in this work.
References
Sato K, Yoshida K, Takahashi S, Anzai JI. pH- and sugar-sensitive layer-by-layer films and microcapsules for drug delivery. Adv Drug Del Rev. 2011;63(9):809–821. | ||
de Villiers MM, Otto DP, Strydom SJ, Lvov YM. Introduction to nanocoatings produced by layer-by-layer (LbL) self-assembly. Adv Drug Del Rev. 2011;63(9):701–715. | ||
Delcea M, Mohwald H, Skirtach AG. Stimuli-responsive LbL capsules and nanoshells for drug delivery. Adv Drug Del Rev. 2011;63(9):730–747. | ||
Gentile P, Carmagnola I, Nardo T, Chiono V. Layer-by-layer assembly for biomedical applications in the last decade. Nanotechnology. 2015;26(42):422001. | ||
De Cock LJ, De Koker S, De Geest BG, et al. Polymeric multilayer capsules in drug delivery. Angew Chem Int Ed. 2010;49(39):6954–6973. | ||
Burke SE, Barrett CJ. pH-dependent loading and release behavior of small hydrophilic molecules in weak polyelectrolyte multilayer films. Macromolecules. 2004;37(14):5375–5384. | ||
Ibarz G, Dahne L, Donath E, Mohwald H. Smart micro- and nanocontainers for storage, transport, and release. Adv Mater. 2001;13(17):1324–1327. | ||
Skirtach AG, Yashchenok AM, Moehwald H. Encapsulation, release and applications of LbL polyelectrolyte multilayer capsules. Chem Commun. 2011;47(48):12736–12746. | ||
Zhao QH, Han BS, Wang ZH, Gao CY, Peng CH, Shen JC. Hollow chitosan-alginate multilayer microcapsules as drug delivery vehicle: doxorubicin loading and in vitro and in vivo studies. Nanomed Nanotechnol Biol Med. 2007;3(1):63–74. | ||
Mao ZW, Ma L, Gao CY, Shen JC. Preformed microcapsules for loading and sustained release of ciprofloxacin hydrochloride. J Control Release. 2005;104(1):193–202. | ||
Gao CY, Donath E, Mohwald H, Shen JC. Spontaneous deposition of water-soluble substances into microcapsules: phenomenon, mechanism, and application. Angew Chem Int Ed. 2002;41(20):3789–3793. | ||
Liu XY, Gao CY, Shen JC, Mohwald H. Multilayer microcapsules as anti-cancer drug delivery vehicle: deposition, sustained release, and in vitro bioactivity. Macromol Biosci. 2005;5(12):1209–1219. | ||
Shen HJ, Shi H, Ma K, et al. Polyelectrolyte capsules packaging BSA gels for pH-controlled drug loading and release and their antitumor activity. Acta Biomater. 2013;9(4):6123–6133. | ||
Shen H, Shi H, Xie M, et al. Biodegradable chitosan/alginate BSA-gel-capsules for pH-controlled loading and release of doxorubicin and treatment of pulmonary melanoma. J Mater Chem B. 2013;1(32):3906–3917. | ||
Vergaro V, Scarlino F, Bellomo C, et al. Drug-loaded polyelectrolyte microcapsules for sustained targeting of cancer cells. Adv Drug Del Rev. 2011;63(9):847–863. | ||
Byeon HJ, Choi SH, Choi JS, et al. Four-arm PEG cross-linked hyaluronic acid hydrogels containing PEGylated apoptotic TRAIL protein for treating pancreatic cancer. Acta Biomater. 2014;10(1):142–150. | ||
Lu B, Xiong SB, Yang H, Yin XD, Zhao RB. Mitoxantrone-loaded BSA nanospheres and chitosan nanospheres for local injection against breast cancer and its lymph node metastases–I: formulation and in vitro characterization. Int J Pharm. 2006;307(2):168–174. | ||
Becker AL, Johnston APR, Caruso F. Layer-by-layer-assembled capsules and films for therapeutic delivery. Small. 2010;6(17):1836–1852. | ||
Anandhakumar S, Nagaraja V, Raichur AM. Reversible polyelectrolyte capsules as carriers for protein delivery. Colloid Surface B. 2010;78(2):266–274. | ||
Skladanowski A, Konopa J. Adriamycin and daunomycin induce programmed cell death (apoptosis) in tumour cells. Biochem Pharmacol. 1993;46(3):375–382. | ||
Aroui S, Brahim S, De Waard M, Breard J, Kenani A. Efficient induction of apoptosis by doxorubicin coupled to cell-penetrating peptides compared to unconjugated doxorubicin in the human breast cancer cell line MDA-MB 231. Cancer Lett. 2009;285(1):28–38. | ||
Eskandani M, Hamishehkar H, Ezzati Nazhad Dolatabadi J. Cyto/Genotoxicity study of polyoxyethylene (20) sorbitan monolaurate (tween 20). DNA Cell Biol. 2013;32(9):498–503. | ||
Eskandani M, Hamishehkar H, Ezzati Nazhad Dolatabadi J. Cytotoxicity and DNA damage properties of tert-butylhydroquinone (TBHQ) food additive. Food Chem. 2014;153:315–320. | ||
del Mercato LL, Rivera-Gil P, Abbasi AZ, et al. LbL multilayer capsules: recent progress and future outlook for their use in life sciences. Nanoscale. 2010;2(4):458–467. | ||
De Koker S, Hoogenboom R, De Geest BG. Polymeric multilayer capsules for drug delivery. Chem Soc Rev. 2012;41(7):2867–2884. | ||
Jones AK, Bejugam NK, Nettey H, Addo R, D’Souza MJ. Spray-dried doxorubicin-albumin microparticulate systems for treatment of multidrug resistant melanomas. J Drug Targeting. 2011;19(6):427–433. | ||
Javier AM, Kreft O, Semmling M, et al. Uptake of colloidal polyelectrolyte-coated particles and polyelectrolyte multilayer capsules by living cells. Adv Mater. 2008;20(22):4281–4287. | ||
Kreft O, Javier AM, Sukhorukov GB, Parak WJ. Polymer microcapsules as mobile local pH-sensors. J Mater Chem. 2007;17(42):4471–4476. | ||
De Geest BG, Vandenbroucke RE, Guenther AM, et al. Intracellularly degradable polyelectrolyte microcapsules. Adv Mater. 2006;18(8):1005–1009. | ||
Shi J, Su Y, Liu W, Chang J, Zhang Z. A nanoliposome-based photoactivable drug delivery system for enhanced cancer therapy and overcoming treatment resistance. Int J Nanomedicine. 2017;12:8257–8275. | ||
Hu C, Gu F, Tai Z, et al. Synergistic effect of reduced polypeptide micelle for co-delivery of doxorubicin and TRAIL against drug-resistance in breast cancer. Oncotarget. 2016;7(38):61832–61844. |
Supplementary materials
© 2018 The Author(s). This work is published and licensed by Dove Medical Press Limited. The full terms of this license are available at https://www.dovepress.com/terms.php and incorporate the Creative Commons Attribution - Non Commercial (unported, v3.0) License.
By accessing the work you hereby accept the Terms. Non-commercial uses of the work are permitted without any further permission from Dove Medical Press Limited, provided the work is properly attributed. For permission for commercial use of this work, please see paragraphs 4.2 and 5 of our Terms.