Back to Journals » Clinical, Cosmetic and Investigational Dermatology » Volume 15

Comparison of the in vitro Anti-Inflammatory Effect of Cannabidiol to Dexamethasone
Authors Wang Y, Wang X, Yang Y, Quan Q, Huo T , Yang S, Ju R, An Q
Received 16 June 2022
Accepted for publication 5 September 2022
Published 16 September 2022 Volume 2022:15 Pages 1959—1967
DOI https://doi.org/10.2147/CCID.S378798
Checked for plagiarism Yes
Review by Single anonymous peer review
Peer reviewer comments 3
Editor who approved publication: Dr Jeffrey Weinberg
Yiming Wang,1– 3 Xue Wang,1– 3 Yang Yang,1– 3 Qianghua Quan,1– 3 Tong Huo,1– 3 Simin Yang,4 Ruijun Ju,4 Quan An1– 3,5
1Research and Development Department, Yunnan Baiyao Group Health Products Co, Ltd, Kunming, People’s Republic of China; 2East Asia Skin Health Research Center, Beijing, People’s Republic of China; 3Research and Development Department, REAL DermaSci & Biotech Co, Ltd, Beijing, People’s Republic of China; 4Beijing Key Laboratory of Enze Biomass Fine Chemicals, Department of Pharmaceutical Engineering, Beijing Institute of Petrochemical Technology, Beijing, People’s Republic of China; 5Research and Development Department, Yunnan Baiyao Group Shanghai Science & Technology Co, Ltd, Shanghai, People’s Republic of China
Correspondence: Quan An, Yunnan Baiyao Group Health Products Co, Ltd, 3686 Yunnan Baiyao Street Chenggong District, Kunming, 650500, People’s Republic of China, Tel +86-13529091004, Email [email protected]
Background: Cannabidiol (CBD) is a non-psychoactive phytocannabinoid constituent of Cannabis sativa with pain-relieving and anti-inflammatory properties. With the emphasis on natural ingredients in cosmetics, CBD has become a new cosmetic ingredient due to its ability to alleviate inflammation. However, in-depth studies that directly compare the effective mechanism and the therapeutic potential of CBD are still needed.
Purpose: The aim of the present study was to investigate the anti-inflammatory effect of CBD in lipopolysaccharide (LPS)-stimulated RAW264.7 macrophages and compare it to dexamethasone (DEX).
Methods: RAW264.7 macrophages in the logarithmic growth phase were incubated in the presence or absence of LPS. After that, the production of nitric oxide (NO), interleukin-6 (IL-6), and tumor necrosis factor-α (TNF-α) were measured. A luciferase reporter assay for nuclear factor kappa B (NF-κB) was performed, and the phosphorylation levels of the mitogen-activated protein kinase (MAPK) and NF-κB signaling pathways were measured.
Results: The present study indicated that CBD had a similar anti-inflammatory effect to DEX by attenuating the LPS-induced production of NO, IL-6, and TNF-α. However, only CBD attenuated JNK phosphorylation levels, and only DEX attenuated IKK phosphorylation levels.
Conclusion: These results suggested that CBD and DEX exhibit similar anti-inflammatory effects on LPS-induced RAW264.7 macrophages mainly through suppressing the MAPK and NF-κB signaling pathways, but with different intracellular mechanisms. These findings suggested that CBD may be considered a natural anti-inflammatory agent for protecting skin from immune disorders.
Keywords: cannabidiol, dexamethasone, LPS, RAW264.7, anti-inflammation
Graphical Abstract:
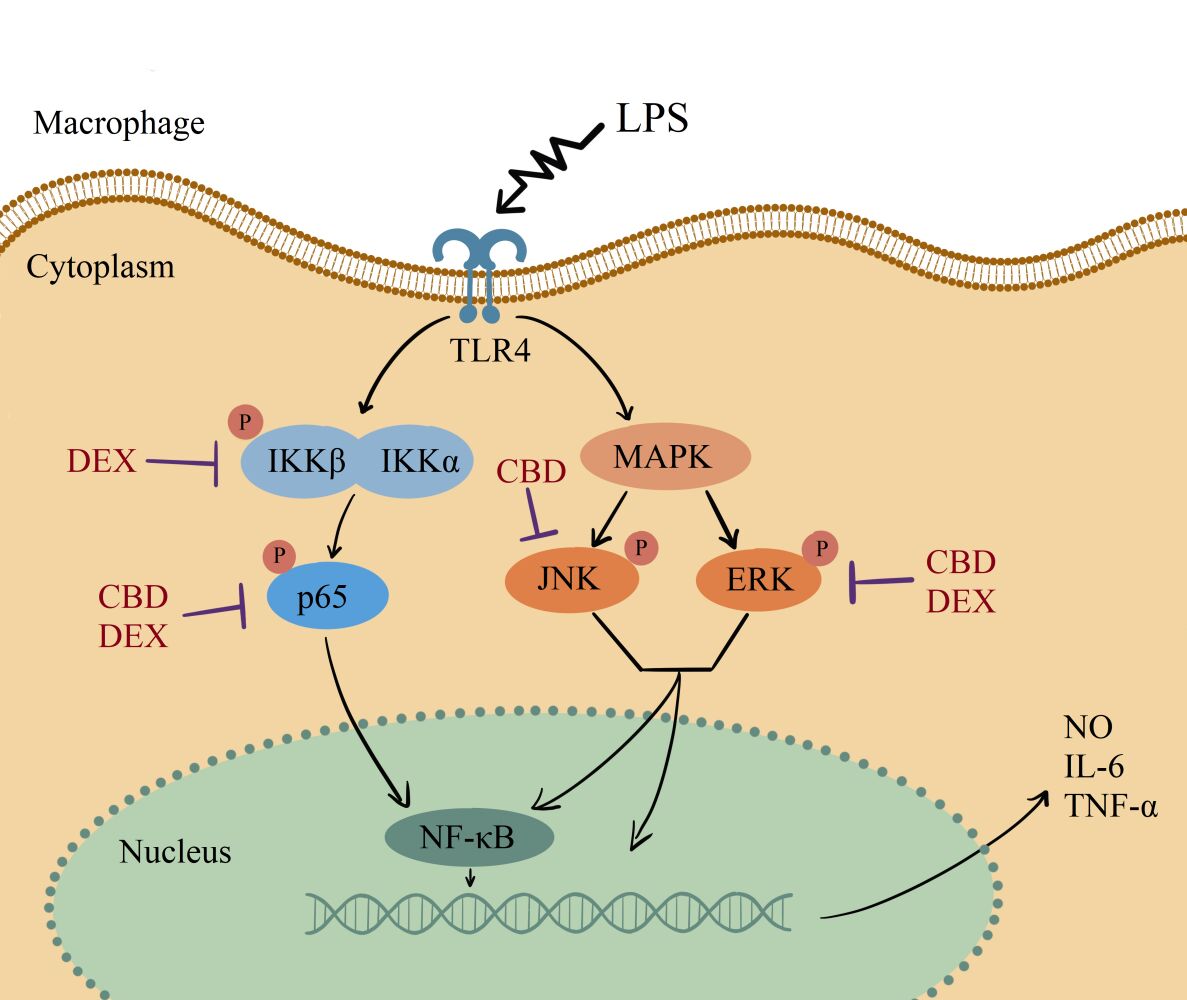
Introduction
Inflammation is a sign of the immune system response to invading pathogens.1 The inflammatory response is connected and highly involved in various diseases such as psoriasis, atopic dermatitis, pancreatitis, and rheumatoid arthritis.2–4 Macrophages, as the main pro-inflammatory cells, play a central role in protection from external intruders. Lipopolysaccharide (LPS) is an outer membrane component of Gram-negative bacteria that induces the release of proinflammatory cytokines.5,6 Several studies have reported that LPS-induced macrophages activate a complex signaling network through toll-like receptor (TLR) including nuclear factor kappa B (NF-κB) and mitogen-activated protein kinase (MAPK) signaling pathway.7 Moreover, the activated macrophages secrete various pro-inflammatory cytokines, such as nitric oxide (NO), interleukin-6 (IL-6), and tumor necrosis factor-α (TNF-α).8,9
Dexamethasone (DEX) is a well-documented immuno-suppressant drugs have benefits patients with inflammation, dermatitis, and allergies.10–12 As a potent glucocorticoid, DEX is well tolerated with most conditions, but it has several adverse effects including weight gain, vomiting, and indigestion.12 In recent years, there has been increasing attention focused on the anti-inflammatory effect of natural compounds due to their minimal side effects.13–15
Cannabidiol (CBD) as the second most prevalent activate component in cannabis, has anti-inflammatory properties in treating various types of inflammation diseases, including airway inflammation, arthritis, intestinal inflammation, and skin inflammation.16–19 The dysregulation of the endocannabinoid system (ECS) in human skin is related to various skin disorders including atopic dermatitis, acne, and hyperpigmentation.20 Recent research found that CBD is able to inhibit the dysregulation of ESC in a dose-dependent manner.21,22 Furthermore, CBD has therapeutic properties to reduce the addictive effects of some drug abuse.23,24 The safe, pain-relieving, and anti-inflammatory properties of CBD have been considered of special interest as a novel therapeutic agent in the dermatology and cosmetics market.25–28
Although there is evidence suggests that the application of CBD may have a similar anti-inflammatory property to other immuone-suppressant drugs for some skin disorders and inflammatory condition, the underlying molecular mechanisms of CBD still need fully identified. The similarities and dissimilarities of their inflammatory response pathways need additional comparative study. The present study aimed to explore the anti-inflammatory mechanism and the therapeutic potential of CBD by comparison to DEX.
Materials and Methods
Reagents
CBD was obtained from Yunnan Baiyao Group Health Products Co., Ltd. The Escherichia coli (O111:B4) LPS was purchased from Sigma-Aldrich (St. Louis, MO, USA). The mouse macrophage cell line RAW264.7 cells and the human colorectal tumor cell lines SW480 cells were purchased from American Type Culture Collection, USA. Dulbecco’s Modified Eagle Medium (DMEM), L-glutamine, penicillin, streptomycin, and fetal bovine serum (FBS) were purchased from EallBio Life Sciences Co., Ltd., Beijing, China. All antibodies in the Western blot were purchased from Abcam. ELISA kits and other chemicals were purchased from Solarbio Life Sciences, Beijing, China.
Cell Culture and Cell Viability
RAW264.7 cells in the logarithmic growth phase were seeded into 6-well cell culture plates at a density of 2×106 cells/mL and incubated overnight at 37°C with 5% CO2. After reaching 70–80% confluency, the supernatant of each well was transferred and incubated in 1mL DMEM supplemented with 100 IU/mL penicillin, 100μg/mL streptomycin, and FBS. CBD was dissolved in dimethyl sulfoxide (DMSO). Subsequently, each well treated with various concentrations of CBD (0.002, 0.004, 0.008, 0.02, 0.04, 0.08, and 0.16 mg/mL) or 0.05 mg/mL DEX. After 6 hours of incubation, 10 μg/mL LPS was supplied for 24 h. Cells treated with vehicle (DMSO) and without LPS were considered as the Control group.
For sulforhodamine-B (SRB) assays, each well was incubated with 100 μL of 0.4% (w/v) SRB, and the plates were shaken for 10 min on an orbital shaker. After that, cells were dissolved in 150 μL of 10 mM Tris base (pH 10.5) for 5 min with shaking. The absorbance at 540 nm was measured on a microplate reader (Biotek, Winooski, VT, USA). The percentage of viable cells is calculated using the following equation:
Measurement of NO, IL-6, and TNF-α Production
RAW264.7 cells were harvested with 0.25% trypsin and maintained in DMEM supplemented with a dissociation solution (0.25% trypsin) and 10% FBS. The cells were seeded into a 96-well plate with a density of 1.2×105 cells/well at 37°C in a 5% CO2 atmosphere for 12 h. After the pellet was treated with 10 μg/mL LPS and with 0.02 mg/mL CBD at 37°C in a 5% CO2 atmosphere for 24 h. For a nitrite assay, each culture medium was mixed with 50μL Griess Reagent I and 50μL Griess Reagent II in a 96-well plate at room temperature. The absorbance of nitrite levels was measured at 540 nm. To determine the effects of CBD and DEX on the production of IL-6 and TNF-α, the supernatant was collected at 100,000 r/min for 10 min at 4°C. The cytokine production in the cell culture medium was measured using an ELISA kit according to the manufacturer’s instructions.
Luciferase Assays
SW480 cells were cultured at 37°C in humidified 5% CO2 conditions until they reaching 70–90% confluence. For cell transfection, reporter DNA was mixed with Lipofectamine 3000 reagent in Opti-MEM at room temperature for 24 h. The generation of stably transfected cell lines were generated by selection and maintained in media containing G418. Stably transfected cells in the logarithmic growth phase were seeded and cultured in 24-well plates at 37°C in a 5% CO2 atmosphere. Cells were then treated with or without CBD and DEX separately for 6 hours. The protein concentration was determined by the BCA protein assay kit (Beijing, China). The luciferase activity was normalized.
Western Blotting
The amount of protein in cells was collected by centrifugation at 1200 rpm at 4°C for 10 min. The concentration of the protein was determined by a BCA assay kit, according to the manufacturer’s instructions. The sample was separated by SDS-PAGE gel electrophoresis. The membranes were blocked with non-fat milk for 30 min and incubated with primary antibodies overnight at 4°C in a shaking incubator. After that, the membranes were probed with specific primary antibodies (JNK, p-JNK, ERK, p-ERK, IKKβ, p-IKKα/β, p65, p-p65, TLR4, and β-actin). Protein bands were visualized using the chemiluminescence detection system (Clinx Company, ChemiScope 6300). The densitometry for the Western blot signal was conducted using the AlphaEaseFC gel-scanning integrated optical density software.
Statistical Analysis
Each experiment was repeated three times. The data were presented as means ± standard deviation (SD). The normality test of all statistical determinations were analyzed by the Shapiro–Wilk normality test. The differences between the results were analyzed by one-way analysis of variance (ANOVA) followed by a Tukey-Kramer test for post hoc comparison. All statistical analyses were performed using GraphPad Prism software. The significant difference compared to control group was indicated by #p<0.05, ##p<0.01, and ###p<0.001. The significant difference compared to the LPS treated group was indicated by * p<0.05, ** p<0.01, and *** p<0.001.
Results
Effect of CBD on RAW264.7 Cell Viability
To assess the cytotoxic effect of CBD, SRB assays were performed in RAW264.7 cells treated with various concentrations of CBD (0.002, 0.004, 0.008, 0.02, 0.04, 0.08, and 0.16 mg/mL). As shown in Figure 1, the RAW264.7 cell viability was decreased in a concentration-dependent manner and inhibited at the doses of 0.04 to 0.16 mg/mL. The concentration of CBD that yielded cell viability higher than 80% was 0.02 mg/mL, therefore that concentration was utilized in subsequent experiments.
![]() |
Figure 1 Effects of CBD on cell viability in RAW264.7 cells induced by LPS. Data are expressed as the mean±SD of three independent experiments. |
The Anti-Inflammatory Effects of CBD and DEX on LPS Stimulated RAW264.7 Cells
To investigate the potential anti-inflammatory effects of CBD and DEX, the NO levels produced by RAW264.7 cells were measured. Compared with untreated cells, the production of NO increased by approximately 5-fold with LPS treatment (Figure 2A). However, the production of NO significantly decreased in the cells pre-treated with CBD and DEX. To compare the inhibitory effects of CBD and DEX on pro-inflammatory cytokines IL-6 and TNF-α, ELISAs were used to evaluate the levels of cytokines released by LPS stimulation of RAW264.7 cells (Figure 2B and C). Treatment with CBD and DEX significantly inhibited the LPS-induced levels of IL-6 and TNF-α.
CBD and DEX Inhibit the LPS-Stimulated NF-κB Signaling Pathway
To elucidate the mechanism of LPS-induced NO production, the expression of luciferase was used to analyze the activation of NF-κB. Compared to the control group, the expression of NF-κB was significantly suppressed with CBD and DEX treatment (Figure 3A). NF-κB is known to transactive inflammatory cytokines to be activated by IKKα/β and p65.29 To further investigate the mechanism of CBD and DEX inhibition of the pro-inflammatory cytokines, the IKKα/β and p65 in LPS-induced RAW264.7 cells were examined by Western blot analysis. As shown in Figure 3B, the level of phosphorylated IKKα/β and p65 were increased with LPS treatment when compared with untreated cells. Figure 3C and D suggest that the anti-inflammatory activity of DEX was due to inhibition of the LPS-induced phosphorylation of IKKα/β and p65, whereas treatment with CBD only inhibited p65 phosphorylation but was not directly related to the phosphorylation of IKKα/β.
CBD and DEX Modulate the MAPK Signaling Pathways in LPS-Induced RAW264.7 Cells
Several research indicated that MAPK also participates in the regulation of NF-κB transcriptional activity through kinase pathways, including JNK and ERK signaling pathways.30,31 To further determine the potential molecular pathways of anti-inflammatory activities of CBD and DEX, the phosphorylated levels of JNK and ERK were examined by Western blot (Figure 4A). The results suggest that the stimulation with LPS increased the phosphorylation levels of JNK and ERK. As shown in Figure 4B and C, CBD-treated cells markedly suppressed the LPS-induced inflammation by activating the phosphorylation of JNK and ERK. DEX also decreased the LPS-induced inflammation by increasing the phosphorylation levels of ERK. However, no inhibitory effects on JNK phosphorylation were observed with pretreated DEX in LPS-induced RAW264.7 cells. Therefore, these findings suggested that CBD modulates the activation of the MAPK pathway through JNK and ERK signaling pathways, whereas DEX only influences the ERK signaling pathway.
Discussion
To further evaluate the mechanisms by which CBD inhibits inflammatory production we examined the LPS-induced macrophage cells pretreated with CBD and compared them to LPS-induced macrophages pretreated with DEX. To establish the inflammatory model, we treated RAW264.7 cells with 10 μg/mL LPS to mimic the inflammatory microenvironment, which allowed the investigation of the anti-inflammatory effect of CBD and DEX. LPS-induced macrophages release various pro-inflammatory mediators, which may cause extensive tissue damage and pathological changes.11,32–34
The inflammatory model was successfully established with LPS stimulation of RAW264.7 cells as indicated by increases in the secretion of the following inflammatory components: NO which is the key pro-inflammatory mediator; IL-6 which is the major pro-inflammatory cytokine; and TNF-α which is the earliest endogenous mediator of inflammatory reaction.35,36 Our results showed that CBD has a similar anti-inflammatory effect to DEX in suppressing the LPS-induced the levels of NO, IL-6, and TNF-α secretion.
We further investigated the signaling pathway involved in the pro-inflammatory induction. Multiple studies regarding LPS induction have suggested that LPS activate the MAPK and NF-κB signaling pathways.37,38 Luciferase assays indicated that the suppression mechanism of CBD and DEX involves the inhibition of NF-κB signaling. However, the pro-inflammatory response patterns of CBD and DEX varied. CBD modulated the MAPK and NF-κB signaling pathways by decreasing the phosphorylation levels of p65, JNK, and ERK, whereas the suppression pattern of DEX involves IKKα/β, p65, and ERK. The phosphorylation level of the p65 subunit was slightly higher in CBD-treated RAW264.7 cells compared to DEX-treated RAW264.7 cells.
These differences probably may attribute to the different effects of CBD and DEX on other activities of RAW264.7 cells. Previous studies have also indicated that CBD, as one of the most abundant active components isolated from Cannabis sativa, can possess the anti-inflammatory effects on LPS-stimulated macrophage cells.39–41 Moreover, the limited clinical comparative study suggested that the topical application of CBD may be efficacious for several inflammatory skin conditions including acne vulgaris and atopic dermatitis.42,43 However, the underlying molecular mechanisms of CBD have yet to be fully identified and the in-depth studies that directly compare the effective mechanism and the therapeutic potential of CBD are still needed.
Inflammation is a complex protective response, including responses from immune cells, to defend the body from harmful agents by triggering a variety of factors.44,45 RAW264.7 cell macrophages, which represent a type of immune cell, has been widely used in the investigation of inflammatory response mechanisms and the etiology of inflammation.46,47 In the present study, CBD suppressed the inflammatory responses by targeting the JNK, whereas DEX suppressed the inflammatory responses by targeting a pathway other than JNK pathway. JNK has been considered a potential therapeutic target for many diseases including skin fibrosis, psoriasis, and skin inflammation.48 Thus, this result suggested that CBD could be valuable for the prevention and treatment of JNK-related inflammatory disease.
Conclusions
As alternative and complementary therapies grow in dermatology, plant extracts such as CBD have garnered significant attention in dermatology. The present study provided new insight of CBD against LPS-induced inflammation. Our results suggested that CBD and DEX suppress the LPS-induced activation of the MAPK and NF-κB signaling pathways in RAW264.7 cells through different intracellular components, indicating that the anti-inflammatory biological mechanism of CBD is different from other immuno-suppressants. Because macrophages exert various pro-inflammatory functions through multiple intracellular pathways, further in vivo and in vitro studies are necessary to enrich the theoretical knowledge of CBD and promote its future clinical application.
Abbreviations
CBD, cannabidiol; DEX, dexamethasone; LPS, lipopolysaccharide; DMSO, dimethyl sulfoxide.
Data Sharing Statement
The authors confirm that the data underlying this article are available within the article.
Acknowledgments
We would like to acknowledge all the participants involved in this study, especially the assistance from the Beijing Key Laboratory of Enze Biomass Fine Chemicals, Beijing Institute of Petrochemical Technology.
Author Contributions
All authors made a significant contribution to the work reported, whether that is in the conception, study design, execution, acquisition of data, analysis, and interpretation, or in all these areas; took part in drafting, revising, or critically reviewing the article; gave final approval of the version to be published; have agreed on the journal to which the article has been submitted; and agree to be accountable for all aspects of the work.
Funding
This work was supported in part by the Yunnan Science and technology project (2018ZF005).
Disclosure
YW, XW, YY, QQ, TH, and QA are employed by the Yunnan Baiyao Group Health Products Co, Ltd. SY and RJ received grants from Yunnan Baiyao Group Health Products Co, Ltd. The authors report no other conflicts of interest in this work.
References
1. Chen L, Deng H, Cui H, et al. Inflammatory responses and inflammation-associated diseases in organs. Oncotarget. 2017;9(6):7204–7218. doi:10.18632/oncotarget.23208
2. Tang J, Diao P, Shu X, Li L, Xiong L. Quercetin and Quercitrin Attenuates the Inflammatory Response and Oxidative Stress in LPS-Induced RAW264.7 Cells: in vitro Assessment and a Theoretical Model. BioMed Res Int. 2019;2019:7039802. doi:10.1155/2019/7039802
3. Gutierrez RMP, Hoyo-Vadillo C. Anti-inflammatory potential of Petiveria alliacea on activated RAW264.7 murine macrophages. Pharmacogn Mag. 2017;13(50):174. doi:10.4103/pm.pm_479_16
4. Lee HN, Shin SA, Choo GS, et al. Anti‑inflammatory effect of quercetin and galangin in LPS‑stimulated RAW264.7 macrophages and DNCB‑induced atopic dermatitis animal models. Int J Mol Med. 2018;41(2):888–898. doi:10.3892/ijmm.2017.3296
5. Fujiwara N, Kobayashi K. Macrophages in inflammation. Curr Drug Targets Inflamm Allergy. 2005;4(3):281–286. doi:10.2174/1568010054022024
6. Aki T, Funakoshi T, Noritake K, Unuma K, Uemura K. Extracellular glucose is crucially involved in the fate decision of LPS-stimulated RAW264.7 murine macrophage cells. Sci Rep. 2020;10(1):10581. doi:10.1038/s41598-020-67396-6
7. Dorrington MG, Fraser IDC. NF-κB Signaling in Macrophages: dynamics, Crosstalk, and Signal Integration. Front Immunol. 2019;4:10.
8. Ju M, Liu B, He H, et al. MicroRNA-27a alleviates LPS-induced acute lung injury in mice via inhibiting inflammation and apoptosis through modulating TLR4/MyD88/NF-κB pathway. Cell Cycle Georget Tex. 2018;17(16):2001–2018. doi:10.1080/15384101.2018.1509635
9. Bode JG, Ehlting C, Häussinger D. The macrophage response towards LPS and its control through the p38(MAPK)-STAT3 axis. Cell Signal. 2012;24(6):1185–1194. doi:10.1016/j.cellsig.2012.01.018
10. Chuang TY, Cheng AJ, Chen IT, et al. Suppression of LPS-induced inflammatory responses by the hydroxyl groups of dexamethasone. Oncotarget. 2017;8(30):49735–49748. doi:10.18632/oncotarget.17683
11. Jain S, Dash P, Minz AP, et al. Lipopolysaccharide (LPS) enhances prostate cancer metastasis potentially through NF-κB activation and recurrent dexamethasone administration fails to suppress it in vivo. The Prostate. 2019;79(2):168–182. doi:10.1002/pros.23722
12. Johnson DB, Lopez MJ, Kelley B. Dexamethasone. StatPearls Publishing; 2022.
13. Ramadan DT, Ali MAM, Yahya SM, El-Sayed WM. Correlation between antioxidant/antimutagenic and antiproliferative activity of some phytochemicals. Anticancer Agents Med Chem. 2019;19(12):1481–1490. doi:10.2174/1871520619666190528091648
14. Guo M, Marek L, Liang Y, Saw PE. Transforming tea catechins into potent anticancer compound: analysis of three boronated-PEG delivery system. Micromachines. 2021;13:1. doi:10.3390/mi13010045
15. Oyenihi AB, Smith C. Are polyphenol antioxidants at the root of medicinal plant anti-cancer success? J Ethnopharmacol. 2019;229:54–72. doi:10.1016/j.jep.2018.09.037
16. Robaina Cabrera CL, Keir-Rudman S, Horniman N, Clarkson N, Page C. The anti-inflammatory effects of cannabidiol and cannabigerol alone, and in combination. Pulm Pharmacol Ther. 2021;69:102047. doi:10.1016/j.pupt.2021.102047
17. De Filippis D, Esposito G, Cirillo C, et al. Cannabidiol reduces intestinal inflammation through the control of neuroimmune axis. PLoS One. 2011;6(12):e28159. doi:10.1371/journal.pone.0028159
18. Lowin T, Tingting R, Zurmahr J, Classen T, Schneider M, Pongratz G. Cannabidiol (CBD): a killer for inflammatory rheumatoid arthritis synovial fibroblasts. Cell Death Dis. 2020;11(8):1–11. doi:10.1038/s41419-020-02892-1
19. Sangiovanni E, Fumagalli M, Pacchetti B, et al. Cannabis sativa L. extract and cannabidiol inhibit in vitro mediators of skin inflammation and wound injury. Phytother Res PTR. 2019;33(8):2083–2093. doi:10.1002/ptr.6400
20. Baswan SM, Klosner AE, Glynn K, et al. Therapeutic potential of cannabidiol (CBD) for skin health and disorders. Clin Cosmet Investig Dermatol. 2020;13:927–942. doi:10.2147/CCID.S286411
21. Bíró T, Tóth BI, Haskó G, Paus R, Pacher P. The endocannabinoid system of the skin in health and disease: novel perspectives and therapeutic opportunities. Trends Pharmacol Sci. 2009;30(8):411–420. doi:10.1016/j.tips.2009.05.004
22. Chye Y, Christensen E, Solowij N, Yücel M. The endocannabinoid system and cannabidiol’s promise for the treatment of substance use disorder. Front Psychiatry. 2019;10:63. doi:10.3389/fpsyt.2019.00063
23. Elsaid S, Kloiber S, Le Foll B. Chapter two - effects of cannabidiol (CBD) in neuropsychiatric disorders: a review of pre-clinical and clinical findings. Rahman S, editor. Progress in Molecular Biology and Translational Science. Vol. 167. Molecular Basis of Neuropsychiatric Disorders: from Bench to Bedside. Academic Press; 2019. 25–75. doi:10.1016/bs.pmbts.2019.06.005
24. Prud’homme M, Cata R, Jutras-Aswad D. Cannabidiol as an intervention for addictive behaviors: a systematic review of the evidence. Subst Abuse Res Treat. 2015;9:33–38. doi:10.4137/SART.S25081
25. Petrosino S, Verde R, Vaia M, Allarà M, Iuvone T, Di Marzo V. Anti-inflammatory properties of cannabidiol, a nonpsychotropic cannabinoid, in experimental allergic contact dermatitis. J Pharmacol Exp Ther. 2018;365(3):652–663. doi:10.1124/jpet.117.244368
26. Maayah ZH, Takahara S, Ferdaoussi M, Dyck JRB. The anti-inflammatory and analgesic effects of formulated full-spectrum cannabis extract in the treatment of neuropathic pain associated with multiple sclerosis. Inflamm Res off J Eur Histamine Res Soc Al. 2020;69(6):549–558. doi:10.1007/s00011-020-01341-1
27. Burstein S. Cannabidiol (CBD) and its analogs: a review of their effects on inflammation. Bioorg Med Chem. 2015;23(7):1377–1385. doi:10.1016/j.bmc.2015.01.059
28. Citti C, Russo F, Linciano P, et al. Origin of Δ9-tetrahydrocannabinol impurity in synthetic cannabidiol. Cannabis Cannabinoid Res. 2021;6(1):28–39. doi:10.1089/can.2020.0021
29. Zhou J, Zhou Q, Zhang T, Fan J. C7ORF41 regulates inflammation by inhibiting NF-κB signaling pathway. BioMed Res Int. 2021;2021:e7413605. doi:10.1155/2021/7413605
30. Schulze-Osthoff K, Ferrari D, Riehemann K, Wesselborg S. Regulation of NF-κB activation by MAP kinase cascades. Immunobiology. 1997;198(1):35–49. doi:10.1016/S0171-2985(97)
31. Alam MB, Chowdhury NS, Sohrab MH, Rana MS, Hasan CM, Lee SH. Cerevisterol alleviates inflammation via suppression of MAPK/NF-κB/AP-1 and activation of the Nrf2/HO-1 signaling cascade. Biomolecules. 2020;10(2):199. doi:10.3390/biom10020199
32. Maldonado RF, Sá-Correia I, Valvano MA. Lipopolysaccharide modification in Gram-negative bacteria during chronic infection. FEMS Microbiol Rev. 2016;40(4):480–493. doi:10.1093/femsre/fuw007
33. Laskin DL, Pendino KJ. Macrophages and inflammatory mediators in tissue injury. Annu Rev Pharmacol Toxicol. 1995;35:655–677. doi:10.1146/annurev.pa.35.040195.003255
34. Li P, Xu Y, Cao Y, Ding Z. Polypeptides isolated from Lactococcus lactis alleviates lipopolysaccharide (LPS)-induced inflammation in Ctenopharyngodon idella. Int J Mol Sci. 2022;23(12):6733. doi:10.3390/ijms23126733
35. Hu K, Yang Y, Tu Q, Luo Y, Ma R. Alpinetin inhibits LPS-induced inflammatory mediator response by activating PPAR-γ in THP-1-derived macrophages. Eur J Pharmacol. 2013;721(1–3):96–102. doi:10.1016/j.ejphar.2013.09.049
36. Sharma JN, Al-Omran A, Parvathy SS. Role of nitric oxide in inflammatory diseases. Inflammopharmacology. 2007;15(6):252–259. doi:10.1007/s10787-007-0013-x
37. Dong J, Li J, Cui L, et al. Cortisol modulates inflammatory responses in LPS-stimulated RAW264.7 cells via the NF-κB and MAPK pathways. BMC Vet Res. 2018;14(1):30. doi:10.1186/s12917-018-1360-0
38. Tabarsa M, Dabaghian EH, You S, et al. The activation of NF-κB and MAPKs signaling pathways of RAW264.7 murine macrophages and natural killer cells by fucoidan from Nizamuddinia zanardinii. Int J Biol Macromol. 2020;148:56–67. doi:10.1016/j.ijbiomac.2020.01.125
39. Khan MI, Sobocińska AA, Czarnecka AM, Król M, Botta B, Szczylik C. The therapeutic aspects of the Endocannabinoid System (ECS) for cancer and their development: from nature to laboratory. Curr Pharm Des. 2016;22(12):1756–1766. doi:10.2174/1381612822666151211094901
40. Monga S, Fares B, Yashaev R, et al. The effect of Natural-Based Formulation (NBF) on the response of RAW264.7 macrophages to LPS as an in vitro model of inflammation. J Fungi. 2022;8(3):321. doi:10.3390/jof8030321
41. Muthumalage T, Rahman I. Cannabidiol differentially regulates basal and LPS-induced inflammatory responses in macrophages, lung epithelial cells, and fibroblasts. Toxicol Appl Pharmacol. 2019;382:114713. doi:10.1016/j.taap.2019.114713
42. Ali A, Akhtar N. The safety and efficacy of 3% Cannabis seeds extract cream for reduction of human cheek skin sebum and erythema content. Pak J Pharm Sci. 2015;28(4):1389–1395.
43. Yuan C, Wang XM, Guichard A, et al. N-palmitoylethanolamine and N-acetylethanolamine are effective in asteatotic eczema: results of a randomized, double-blind, controlled study in 60 patients. Clin Interv Aging. 2014;9:1163–1169. doi:10.2147/CIA.S65448
44. Medzhitov R. Origin and physiological roles of inflammation. Nature. 2008;454(7203):428–435. doi:10.1038/nature07201
45. Arulselvan P, Fard MT, Tan WS, et al. Role of antioxidants and natural products in inflammation. Oxid Med Cell Longev. 2016;2016:5276130. doi:10.1155/2016/5276130
46. Elisia I, Pae HB, Lam V, Cederberg R, Hofs E, Krystal G. Comparison of RAW264.7, human whole blood and PBMC assays to screen for immunomodulators. J Immunol Methods. 2018;452:26–31. doi:10.1016/j.jim.2017.10.004
47. Wang X, Hu X, Yan H, Ma Z, Deng X. Pro-inflammatory effects of a litchi protein extract in murine RAW264.7 macrophages. Hortic Res. 2016;3(1):1–7. doi:10.1038/hortres.2016.17
48. Hammouda MB, Ford AE, Liu Y, Zhang JY. The JNK signaling pathway in inflammatory skin disorders and cancer. Cells. 2020;9(4):857. doi:10.3390/cells9040857
© 2022 The Author(s). This work is published and licensed by Dove Medical Press Limited. The full terms of this license are available at https://www.dovepress.com/terms.php and incorporate the Creative Commons Attribution - Non Commercial (unported, v3.0) License.
By accessing the work you hereby accept the Terms. Non-commercial uses of the work are permitted without any further permission from Dove Medical Press Limited, provided the work is properly attributed. For permission for commercial use of this work, please see paragraphs 4.2 and 5 of our Terms.