Back to Journals » OncoTargets and Therapy » Volume 17

Amplifying Curcumin’s Antitumor Potential: A Heat-Driven Approach for Colorectal Cancer Treatment
Authors Kabagwira J, Fuller RN , Vallejos PA, Sugiono CS, Andrianarijaona VM, Chism JB, O'Leary MP, Caba Molina D, Langridge W, Senthil M, Wall NR
Received 10 November 2023
Accepted for publication 25 January 2024
Published 30 January 2024 Volume 2024:17 Pages 63—78
DOI https://doi.org/10.2147/OTT.S448024
Checked for plagiarism Yes
Review by Single anonymous peer review
Peer reviewer comments 2
Editor who approved publication: Dr Arseniy Yuzhalin
Janviere Kabagwira,1,2 Ryan N Fuller,1,2 Paul A Vallejos,1,2 Chase S Sugiono,1 Vola-Masoandro Andrianarijaona,1 Jazmine Brianna Chism,2 Michael P O’Leary,3 David Caba Molina,3 William Langridge,1,2 Maheswari Senthil,4 Nathan R Wall1,2
1Department of Basic Science, Division of Biochemistry, Loma Linda University, Loma Linda, CA, USA; 2Center for Health Disparities and Molecular Medicine, Loma Linda University, Loma Linda, CA, USA; 3Division of Surgical Oncology, Department of Surgery, Loma Linda University Health, Loma Linda, CA, USA; 4Division of Surgical Oncology, Department of Surgery, Irvine Medical Center, University of California, Orange, CA, USA
Correspondence: Nathan R Wall, Department of Basic Science, Division of Biochemistry, Loma Linda University, 11085 Campus Street, Mortensen Hall, Room 162, Loma Linda, CA, 92350, USA, Tel +1-909-558-4000 x81397, Fax +1-909-558-0177, Email [email protected]
Introduction: Peritoneal metastases from colorectal cancer (CRC) present a significant clinical challenge with poor prognosis, often unresponsive to systemic chemotherapy. Cytoreductive surgery (CRS) combined with hyperthermic intraperitoneal chemotherapy (HIPEC) is a treatment approach for select patients. The use of curcumin, a natural compound with antitumor properties, in HIPEC is of interest due to its lower side effects compared to conventional drugs and potential for increased efficacy through direct delivery to the peritoneal cavity.
Methods: An in vitro hyperthermic model was developed to simulate clinical HIPEC conditions. Three colon cancer cell lines (SK-CO-1, COLO205, SNU-C1) representing different genetic mutations (p53, KRAS, BRAF) were treated with either curcumin (25 μM) or mitomycin-C (1 μM) for 1, 2, or 3 hours. Post-treatment, cells were incubated at 37°C (normothermia) or 42°C (hyperthermia). Cell viability and proliferation were assessed at 24, 48 and 72 hours post-treatment using Annexin V/PI, MTT assay, trypan blue exclusion, and Hoffman microscopy.
Results: Hyperthermia significantly enhanced the antitumor efficacy of curcumin, evidenced by a two-fold reduction in cell viability compared to normothermia across all cell lines. In the SNU-C1 cell line, which harbors a p53 mutation, mitomycin-C failed to significantly impact cell viability, unlike curcumin, suggesting mutation-specific differences in treatment response.
Discussion: The findings indicate that hyperthermia augments the antitumor effects of curcumin in vitro, supporting the hypothesis that curcumin could be a more effective HIPEC agent than traditional drugs like mitomycin-C. Mutation-associated differences in response to treatments were observed, particularly in p53 mutant cells. While further studies are needed, these preliminary results suggest that curcumin in HIPEC could represent a novel therapeutic strategy for CRC patients with peritoneal metastases. This approach may offer improved outcomes with fewer side effects, particularly in genetically distinct CRC subtypes.
Plain Language Summary: In this study, we aimed to improve treatments for peritoneal carcinomatosis (PC), a challenging form of colorectal cancer (CRC) that does not respond well to regular chemotherapy. We explored an approach called hyperthermic intraperitoneal chemotherapy (HIPEC), which involves delivering potent chemotherapy directly to the abdominal area at an elevated temperature. Our innovative idea was to enhance HIPEC’s effectiveness by using curcumin, a natural compound with fewer side effects than conventional HIPEC drugs. To test our theory, we conducted experiments on colon cancer cells under high-temperature conditions, mimicking what occurs during HIPEC treatments. We also compared curcumin with the standard drug, mitomycin-C (MMC). We discovered that curcumin, when delivered under high temperatures, outperformed MMC in killing colorectal cancer cells. This is an exciting breakthrough because it suggests that curcumin in HIPEC could be a promising alternative for treating PC. While we are still working on more comparisons and further studies, this outcome represents a significant step towards better treatment options for patients with peritoneal carcinomatosis. In conclusion, our study was motivated by the need to find more effective ways to combat an aggressive form of colorectal cancer metastasis. By exploring the potential of curcumin in HIPEC, we hope to provide better treatment options for patients who face this challenging disease.
Keywords: peritoneal carcinomatosis, curcumin, hyperthermic, colorectal, mitomycin-C
Graphical Abstract:
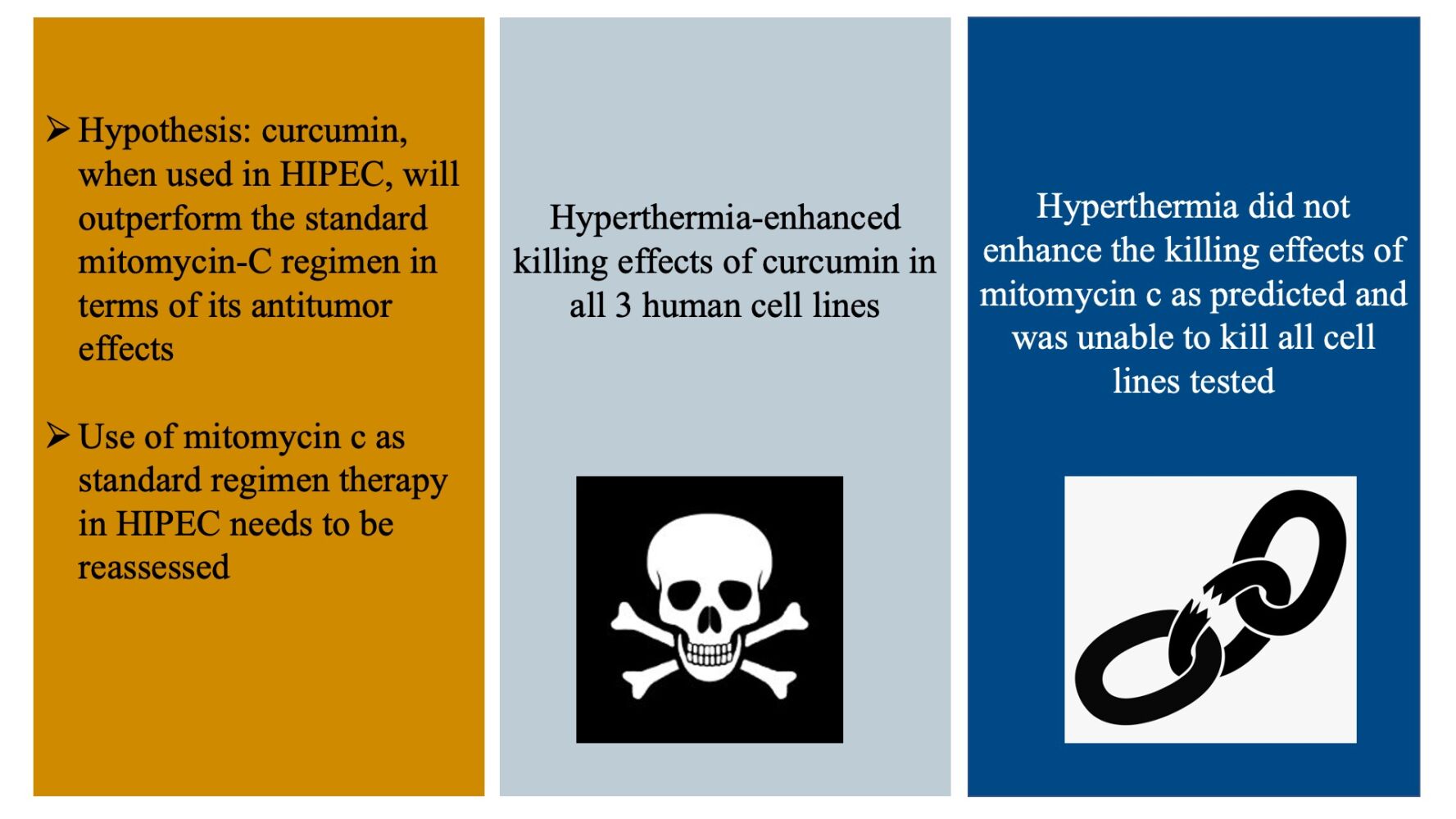
Introduction
Peritoneal dissemination from colorectal and appendiceal neoplasms pose significant therapeutic challenges and survival outcomes is dismal relative to other colorectal metastatic disease with standard treatment.1 Peritoneal carcinomatosis (PC) from colorectal cancer is detected in 10–15% of cases at the time of original presentation and 30–40% in the recurrence setting2 with a median survival of 5 months if untreated.3 Cytoreductive surgery (CRS) with Hyperthermic Intraperitoneal Chemotherapy (HIPEC) is considered a therapeutic option in appropriately selected patients with PC and has been shown to improve survival.4 Patient outcomes from PC after adequate cytoreduction vary widely as the risk of peritoneal recurrence and systemic metastases vary significantly. Cytoreductive surgery is an extensive surgical treatment with high morbidity.5 Patients who are likely to develop early recurrence after cytoreduction may not benefit from this procedure. Prognosticators such as peritoneal cancer index (PCI), which is an assessment of the extent of peritoneal dissemination, and type of peritoneal spread (tumor grade), are obtained during surgery.6 Serum markers that are predictive of prognosis are lacking. Furthermore, the response of chemotherapeutic agents by PC is poor,7 demanding a more critical look at the biological mechanisms that contribute to the development and progression of PC thus surgical treatment with cytoreduction and HIPEC has not been widely standardized.
Beyond HIPEC and systemic therapy, there are other treatment modalities for managing peritoneal carcinomatosis from colorectal cancer. One notable alternative is Pressurized Intraperitoneal Aerosol Chemotherapy (PIPAC), a minimally invasive approach that delivers pressurized chemotherapy in the form of an aerosol directly into the peritoneal cavity.8,9 This method allows for high local drug concentration with minimal systemic absorption, potentially reducing systemic toxicity. PIPAC has shown promise in improving drug penetration and distribution in the peritoneum,10 making it a suitable option for patients who are not candidates for HIPEC. Other therapeutic strategies include palliative care focusing on symptom management, intraperitoneal radiotherapy,11 and emerging targeted therapies12 that address specific molecular pathways involved in tumor growth and spread. In cases where PC is limited or resectable, surgical debulking or peritonectomy might be considered to reduce tumor load.13,14 It is important to note that the choice of treatment is highly individualized, depending on factors such as the extent of disease spread, patient’s overall health, and specific tumor characteristics. The development and optimization of these varied therapeutic approaches are critical for expanding treatment options and improving outcomes for patients with peritoneal carcinomatosis.
Intraperitoneal chemotherapy is administered for the treatment of PC because the peritoneal-plasma barrier allows for higher drug concentrations to be used which results in a higher efficacy with lower systemic toxicities.15 Although there is strong evidence to demonstrate improved survival with cytoreductive surgery,4 the role of hyperthermic conditions has been questioned16 given the heterogeneity of the studies and needs further evaluation. Despite optimal cytoreduction, a subset of patients will suffer early peritoneal recurrence which indicates that intraperitoneal chemotherapy was ineffective in controlling the microscopic disease.17 In vitro studies focused on optimizing the drug, duration of exposure, and temperature, based on the biology of the cancer, are critically needed to inform the design of future clinical trials.
Mitomycin-C (MMC) is currently the most common and standard perfusion agent in the United States.18 Though several other chemotherapeutic agents such as cisplatin, oxaliplatin, carboplatin, doxorubicin, 5-fluorouracil and irinotecan have been evaluated in HIPEC, none has been described as being superior.19,20 Therefore, the focus of the work presented in this manuscript is to evaluate the turmeric derivative curcumin under hyperthermic conditions.
Curcumin has been considered as a potential anticancer therapy due to its anti-inflammatory effects and capacity to interrupt signaling pathways crucial for cancer initiation and progression.21,22 Moreover, the negligible toxicity makes curcumin a safe therapeutic, including cancer treatment.23,24 Preclinical studies involving curcumin in colorectal cancer have shown that curcumin enhances 5-Fluorouracil sensitivity in vitro and in vivo.24,25 In addition, Phase I and II clinical trials have yielded promising results on the use of curcumin as part of colorectal cancer therapeutic strategies.26–29 Specifically for PC, curcumin prepared in polymeric micelles has been identified to possess higher inhibitory effects against tumors in a colorectal peritoneal carcinomatosis mouse model in which tumor growth and metastasis were suppressed and survival of the tumor-bearing mice was prolonged.30,31
Although there is strong evidence to demonstrate improved survival with CRS, the role of HIPEC needs further evaluation. Despite optimal cytoreduction, a subset of patients suffers early peritoneal recurrence which indicates that intraperitoneal chemotherapy was ineffective in controlling the microscopic disease. In vitro studies focused on the efficacy of curcumin; namely, duration of exposure, and temperature. These parameters are critically needed to inform the design of future clinical trials. Here, we demonstrate in three human colon cancer cell lines, that curcumin under HIPEC-like conditions, resulted in increased overall killing in comparison to MMC, which was significantly less effective in killing cells harboring a mutation in the tumor suppressor gene p53.
Methods
Cell Culture
The CT26 mouse colon cancer cell line was a kind gift from Wilbur B. Bowne, MD, Department of Surgery, Drexel University College of Medicine. CT26 cell line was authenticated using Short Tandem Repeat (STR) analysis as described in the National Institute of Standards and Technology (NIST) granted US patent (No. 9,556,482) (ATCC, Manassas, VA). COLO205, SK-CO-1 and SNU-C1 colon cancer cell lines derived from ascites, peritoneum and ascites, respectively, were purchased from American Type Culture Collection (ATCC, Manassas, VA). The COLO205 cell line has mutations in APC, p53, BRAF and SMAD4, SK-CO-1 has reported mutations in APC and KRAS, and SNU-C1 has a known p53 mutations (Table 1). COLO205, SNU-C1 and CT26 cells were maintained in RPMI 1640 (Cellgro, Mediatech, Inc., Manassas, VA) supplemented with 10% heat inactivated FBS, 100 units of penicillin, 100 µg/mL of streptomycin, and 100 µg/mL L-glutamine (Corning, Mediatech, Inc, Manassas, VA). SK-CO-1 cells were maintained in EMEM (Quality Biological, Gaithersburg, MD) supplemented with 10% heat inactivated FBS, 100 units of penicillin, 100 µg/mL of streptomycin, and 100 µg/mL L-glutamine (Corning, Mediatech, Inc, Manassas, VA). Cells were all originally cultured at 37°C in a humidified incubator with 5% CO2.
![]() |
Table 1 Disease Characteristics of Three Human and One Mouse Colon Cancer Cell Lines |
Short Tandem Repeat (STR) Analysis
CT26 cells were authenticated using STR (ATCC, Manassas, VA). Eighteen Mouse short tandem repeat (STR) loci were analyzed. Two additional markers (Human D8 and D4) to screen for the presence of human or African green monkey species were also utilized. Each sample was processed using the ABI Prism® 3500xl Genetic Analyzer. Data were analyzed using GeneMapper® ID-X v1.2 software (Applied Biosystems). Appropriate positive and negative controls were run and confirmed for each assay set.
Preparation of Solutions
Curcumin (Sigma Aldrich, St. Louis, MO) stock solutions were prepared (13.5mM) using 5.4% DMSO and 94.6% ethanol as solvents and used not more than twice after storage at −20°C. Subsequent dilutions were made from the stock solution in fully supplemented RPMI 1640 or EMEM to a final concentration of 10, 25 and 50 µM. MMC [1mg/mL] was a kind gift from Loma Linda Cancer Center Pharmacy and was administered at a final concentration of 1µM and was prepared by diluting the stock solution in fully supplemented cell culture medium. For experiments with 0µM Curcumin, the respective diluent, DMSO (Dimethyl Sulfoxide) and ethanol was added to achieve the desired concentration. Both diluents, DMSO and ethanol, were of analytical grade and free from any contaminants that could interfere with the experiments.
Treatment and Hyperthermic Experiments and Viability Assay
For the short term hyperthermic experiments, CT26, COLO205, SNU-C1 and SK-CO-1 cells were seeded at a density of 0.5×105 cells/mL in a 12 well plate and allowed to attach overnight. Cells were then placed at either 37°C or 42°C for 1 or 1.5, 3, or 6 hours after which cells were trypsinized and evaluated for cell death using Trypan blue exclusion reagent protocols (Life Technologies, Grand Island, NY). Viability and cell number was evaluated on 50,000 total cells using a TC20 Automated Cell Counter with dual chamber counting slides (BioRad, Hercules, CA). For long duration experiments, cells were treated with a 10, 25 or 50 μM final concentration of curcumin or 1µM MMC and placed at either 37°C or 42°C for 1, 2, or 3 hours, after which media containing MMC or curcumin treatment was replaced with new medium devoid of treatment drugs. Cells were then incubated at 37°C for an additional 24, 48 or 72 hours as it is illustrated in Figure 1A. Cells were trypsinized and evaluated for cell death using Trypan blue exclusion reagent protocols (Life Technologies, Grand Island, NY). Viability and cell number was evaluated on 50,000 total cells using a TC20 Automated Cell Counter with dual chamber counting slides (BioRad, Hercules, CA).
Annexin V Apoptosis and Flow Cytometry
Cells were washed with 200 µL PBS and resuspended in 100μL staining buffer (FITC Annexin V Apoptosis Detection Kit with PI, BioLegend, San Diego, CA) by mixing 2μL of Annexin-V and 5μL propidium iodide (PI) in 1x Annexin V binding buffer (FITC Annexin V Apoptosis Detection Kit with PI, BioLegend, San Diego, CA) according to manufacturer’s instructions. The cells were incubated for 30 min at room temperature and protected from light. After centrifugation at 1500 rpm for 5 min, the staining buffer was aspirated, and the cells were washed in 200μL PBS. Samples were fixed in 1% PFA prior to analysis. For cell cycle analysis cells were harvested, prepared and analyzed for DNA content as previously reported.32 Flow cytometry analysis was performed using a MACSQuant analyzer (Miltenyi Biotec Inc., Auburn, CA, USA) and FlowJo analysis software (v.10.9) (FlowJo, Ashland, OR, USA).
Methyl Thiazolyl Tetrazolium (MTT) Assay
MTT powder was dissolved in PBS at a concentration of 25mg/mL, sterilized through a 0.22 μm filter and stored in the dark at 4°C until use. Cells were cultured as above in a 96-well microplate at a seeding density of 5000 cell per well. Cells were left to attach overnight and then treated with either 10, 25, or 50 μM curcumin or 0.25, 0.5, or 1 μM MMC. After 72h of treatment, culture medium was removed, and the cells were washed with PBS. Fresh culture medium (100 μL) was added to each well of the microplate along with 50 μL of MTT solution. The microplate was then incubated at 37°C and 5% CO2 for 3 hours. Plates were next spun at 500xg for 5 min, and medium was carefully removed from each well in order to not disturb the MTT formazan crystals that formed at the bottom of each well. 100 μL of DMSO was added to each well, and the microplate was gently agitated for 15 min to dissolve the MTT formazan crystals after which the absorbance of the samples was read at 570 nm using a plate reader with a reference wavelength of 630 nm. The absorbance is proportional to the number of viable cells in each well.
Statistical Analysis
Student’s paired one-tailed t-test was performed on the data from these experiments. Statistical analyses were performed using RStudio software. A probability of <0.05 was considered to be significant. Data are presented as the mean and standard deviation and are representative of at least 3 independent experiments.
Results
Assessing the Effect of Short-Term Heat Exposure of the CT26 Mouse Colon Cell Line
Cells were first assessed to determine the effect of short-term hyperthermic exposure to 42°C. Mouse CT26 colon cells were placed at either 37°C or 42°C for 1.5, 3, or 6 hours, after which cells were incubated at 37°C for an additional 24, 48 or 72 hours as illustrated in Figure 1A. Cells were then trypsinized and evaluated for cell death using Trypan blue exclusion reagent protocols (Life Technologies, Grand Island, NY). Viability and cell number was evaluated on 50,000 total cells using a TC20 Automated Cell Counter with dual chamber counting slides (BioRad, Hercules, CA). The heat did not have a significant effect on the CT26 mouse cell viability for up to 3 hours (Figure 1B) but did result in near 50% killing after 6 hours at 42°C. CT26 mouse cells were used along with human-derived cell lines as a mouse model of HIPEC treatment is under development in our labs.
Assessing the Effect of Short-Term Heat Exposure of the Human Colon Cell Lines COLO205, SK-CO-1 and SNUC-1
As with the CT26 mouse cells, human cancer cell lines were assessed to determine the short-term effects of heat (42°C) exposure and viability. Human colon cells (COLO205, SK-CO-1 and SNUC-1) were placed at either 37°C or 42°C for 1, 3, or 6 hours. Cells were trypsinized and evaluated for cell death using Trypan blue exclusion reagent protocols (Life Technologies, Grand Island, NY). Viability and cell number was evaluated on 50,000 total cells using a TC20 Automated Cell Counter with dual chamber counting slides (BioRad, Hercules, CA). The heat did not have a significant effect on the cell viability of these human cells for up to 6 hours (Figure 1C).
Curcumin Dosage and Time Course Effects on CT26 Mouse Colon Cancer Cells
CT26 mouse cells were seeded at a density of 1×105 cells/mL/well in 12 well plates and allowed to attach overnight. The cells were then treated with curcumin to final concentrations of 0 µM, 10 µM, 25 µM and 50 µM for 1, 2, and 3 hours. Curcumin was dissolved in ethanol and DMSO in a ratio of 94.6% and 5.4%, respectively, to make the stock solution. The cells exposed to curcumin were assessed for viability using Trypan blue exclusion as above after 72 hours at each time point Figure 2 and we observed a time- and dose-dependent decrease in cellular proliferation and viability (Figure 2A–C) by using Hoffman microscopy. Furthermore, quantitation of the CT26 cells treated with curcumin for 3 hours under normothermic and hyperthermic conditions showed that after 24, 48 and 72 hours of incubation, there were near-immediate measurable reductions in cell numbers in curcumin-treated cells after 24 hours that became more significant with increased time and dose (Figure 2D). These findings highlight the potential benefits of combining curcumin with hyperthermia therapy in the treatment of colon cancer.
Dose and Time Course Effects of Curcumin and MMC on Human Colon Cancer Cells
Human colon cancer cells were again seeded at a density of 1×105 cells/mL/well in 12 well plates and allowed to attach overnight. Cells were then treated with curcumin to final concentration of 25 µM or 1µM MMC for 1, 2, and 3 hours. The cells exposed to curcumin or MMC were assessed for viability using Trypan blue exclusion as above after 24, 48 and 72 hours at each time point and we observed a time- and dose-dependent decrease in cellular proliferation and viability (Supplemental Figures 1 and 2. In Figure 3 we specifically show the hyperthermia-enhanced killing effects of curcumin in all three cell lines at 1, 2, and 3 hours (Figure 3A) as measured after 72 hours of follow-on incubation. Hyperthermia enhanced the killing properties of MMC in the COLO205 and SK-CO-1 cells but unlike curcumin was unable to kill the SNU-C1 cell line under normothermic or hyperthermic conditions (Figure 3B) indicating that curcumin may produce a more general killing ability when combined with hyperthermic temperatures in these cells than the standard regimen MMC modality. This specific finding that hyperthermic conditions enhance curcumin and MMC killing ability in COLO205 and SK-CO-1 cells while only curcumin killing of SNU-C1 cells is enhanced when incubated for 2 and 3 hours at 42°C is simplified in Figure 3C. Figure 3 Continued.
Assessing Apoptosis Levels in Curcumin and MMC Treatment Under Hyperthermic Conditions
To assess whether hyperthermia-induced reduction in cell number was due to cell death from apoptosis or necrosis, cells were treated with 25 µM curcumin or with 1 µM MMC and incubated at either 37°C or 42°C for two hours then incubated at 37°C as illustrated in Figure 1A. Seventy-two hours later, cells were harvested and stained with propidium iodide (PI) and annexin V to assess apoptosis using flow cytometry performed using a MACSQuant analyzer (Miltenyi Biotec Inc., Auburn, CA, USA) and FlowJo analysis software (FlowJo, Ashland, OR, USA). The results for curcumin treatments show a high percentage of early and late apoptosis in the cells treated under hyperthermia for COLO205 and SK-CO-1 cell lines compared with those treated under normothermia (Figure 4). MMC affected the COLO205 and SK-CO-1 cells equally with no enhancement in apoptotic or necrotic cells measured as a result of hyperthermic conditions (Figure 4). In this annexin V/PI study, no killing affect in SNU-C1 cells was measured after curcumin or MMC treatment (Figure 4).
Human colon cancer cells (COLO205, SNU-C1 and SK-CO-1) were next incubated under either 37°C (normothermia) or 42°C (hyperthermia) conditions for 3 hours with either curcumin (Figure 5A) or MMC (Figure 5B). Cell cultures were moved to 37°C (time 0) for 72 hours. Cells were then stained for MTT and analyzed using spectrophotometry. For curcumin treatment (Figure 5A), the three cell lines were treated with 10, 25 and 50 μM of curcumin while with MMC treatments (Figure 5B) the cell lines were treated with 0.25, 0.5 and 1 μM. All doses of curcumin (Figure 5A) under hyperthermic conditions resulted in absorbance readings being reduced as compared to normothermic conditions. The greatest absorbance reduction difference was measured in COLO205 and SK-CO-1 cells treated with 25 µM curcumin. MMC treatment (Figure 5B) of COLO205 and SK-CO-1 resulted in reduced absorbance readings in both normothermic and hyperthermic conditions with significant advantage provided by the increased temperature. No significant observable reductions were measured in the SNU-C1 cells after MMC treatment under hyperthermic conditions, and no significant difference was measured in either the curcumin or MMC treatments (Figure 5B).
Discussion
HIPEC presents a promising treatment option for peritoneal carcinomatosis compared to systemic chemotherapy alone due to the low penetration of systemic chemotherapy in the peritoneal cavity.33 Additionally, HIPEC has been shown to improve the result of systemic chemotherapy compared to systemic chemotherapy alone.33 Relative to systemic therapy, the peritoneal cavity is thought to be more resistant to chemotherapy due to poor blood circulation. However, this characteristic is beneficial for the use of HIPEC treatment in that it increases intraperitoneal bioavailability without increasing systemic toxicity and allows for a prolonged exposure of chemotherapy. Low systemic blood circulation facilitates the use of high therapeutic doses in the peritoneal cavity with minimal toxicity.34 The goal of HIPEC is to maximize tumor penetration and optimize cell death while minimizing systemic toxicity. Unfortunately, few chemotherapeutics have been optimized for use under HIPEC conditions35 and not all agents that work for systemic chemotherapy work for HIPEC.
Finding an agent that would provide a better solution without the morbidity associated with standard chemotherapeutic regimens is an ongoing research endeavor. One such agent may be curcumin, a polyphenolic compound found in the yellow spice turmeric. Curcumin has been found to possess anti-inflammatory,36 anti-angiogenesis,37,38 anti-cancer39,40 and anti-oxidative41 properties. It has been studied as a form of treatment42–44 for various cancer types, including highly treatment-resistant cancers.45,46 Preliminary trials have shown promise in improving survival rates and reducing morbidity and mortality associated with cancer surgeries.47–49
Interestingly, curcumin, derived from turmeric, is widely recognized for its potent anti-inflammatory properties.50,51 These effects are mediated through its ability to modulate various molecular targets involved in inflammation, such as cytokines, transcription factors, and enzymes like cyclooxygenase-2 (COX-2)22,52 and lipoxygenase.29 In the context of cancer, inflammation plays a dual role. Chronic inflammation is known to contribute to tumor progression,29 providing a rationale for using anti-inflammatory agents like curcumin in cancer therapy. By reducing inflammation, curcumin can potentially inhibit the tumor-promoting environment that chronic inflammation creates.
However, recent studies have highlighted a complex scenario where acute, pro-inflammatory responses, particularly those leading to a specific type of cell death known as immunogenic cell death (ICD), can be advantageous in cancer treatment.53–55 ICD not only destroys cancer cells but also induces an immune response against them.56 This process is characterized by the release of damage-associated molecular patterns (DAMPs), which can activate the immune system to recognize and attack tumor cells.57
In the treatment of PC, this dual role of inflammation presents a unique therapeutic challenge. While curcumin’s anti-inflammatory action can help in mitigating the chronic inflammatory environment conducive to tumor growth, there is also a need to harness the potential benefits of pro-inflammatory responses associated with ICD. This suggests a potential therapeutic strategy where curcumin could be used in combination with treatments that promote ICD. The goal would be to strike a balance – reducing harmful inflammation while simultaneously eliciting a robust anti-tumor immune response.
Given this complexity, it is crucial to further explore the relationship between curcumin’s anti-inflammatory properties and the role of pro-inflammatory cell death in PC. More research is needed to understand how curcumin can be optimally used in PC treatment, potentially in combination with other therapies, to leverage both its anti-inflammatory effects and the beneficial aspects of a pro-inflammatory response. This could lead to more effective and targeted therapeutic strategies for managing peritoneal carcinomatosis.
This study was initiated to determine if the killing effects of curcumin could be enhanced under hyperthermic conditions, thus overcoming some of the bioavailability issues this agent has due to its nature. Our study confirmed that 42°C perfusion is not cytotoxic to the human cells for up to 6 hours giving us the impetus to test three short-term duration exposures of colon cancer cell lines under that temperature. MMC was used as a control as it is commonly used in HIPEC treatment of peritoneal carcinomatosis.58–61 For MMC, exposing cancer cells to hyperthermia in vitro resulted in killing which started after 1 hour of hyperthermia (Supplemental Figure 2A–C) while for curcumin the cytotoxic effects were observed after two hours (Supplemental Figure 1D–F). We observed that the cytotoxicity of MMC was not enhanced by hyperthermic conditions and did not kill all the cell lines equally (Supplemental Figure 2). In fact, SNU-C1, the cell line derived from human peritoneum, and thus, most similar to peritoneal carcinomatosis, was not affected by hyperthermia combined with MMC.
Of note, the SNU-C1 cell line carries a mutated p53 protein. The p53 tumor suppressor protein plays a critical role in the regulation of DNA repair and apoptosis.62,63 When DNA damage occurs, p53 initiates DNA-repair mechanisms.64,65 In the case of a mutant p53 carrying cell line, the response to MMC-induced DNA damage is compromised as is the attenuated cell killing response seen after MMC treatment. The response of a p53 mutant cell line to MMC can vary depending on the specific mutation or mutations and the cellular context and perhaps is the reason COLO205, which also has a p53 mutation, is still responsive to MMC with and without hyperthermia. Other factors such as the presence of compensatory genetic alterations or alternative survival pathways may also influence the sensitivity of the cell line to MMC and should be investigated. The efficacy of curcumin was enhanced by hyperthermic conditions in a statistically significant manner (Figures 3–5) even in the SNU-C1 cells. Curcumin’s killing mechanism on COLO205 and SK-CO-1 cells can be characterized as apoptotic, while its killing mechanism in the SNU-C1 cells is not. Rather, an autophagic or senescence-enhancing mechanism has been proposed with this work still ongoing in the lab. In all cell lines tested, hyperthermia combined with curcumin did result in the most enhanced cell death as compared to the standard regimen therapeutic MMC. In addition, MMC’s ability to kill cancer cells could be limited by the genotype of these cells where curcumin did not appear to have these same limitations. This study underscores a critical consideration regarding the choice of HIPEC agents for the treatment of PC, revealing that MMC is not uniformly effective in killing all cancer cells, especially those with specific genetic mutations. The ethical implications of using MMC as the preferred HIPEC-enhancing agent, given its limitations, warrant careful evaluation.
The intraperitoneal administration of chemotherapy agents in HIPEC can cause chemotherapy-related toxicities.66–68 These factors may include bone marrow suppression, gastrointestinal toxicity (nausea, vomiting, diarrhea), liver and kidney dysfunction, electrolyte imbalances, and hematological abnormalities.69,70 The specific toxicities can vary depending on the agents used in the HIPEC protocol. Hyperthermia itself can cause heat-related risks, as it involves heating the abdominal cavity to temperatures between 41–43°C (105–109°F). These elevated temperatures can lead to thermal injury to normal tissues, including the intestines, kidneys, and liver. It may also result in metabolic changes, such as electrolyte imbalances and fluid shifts.71–74 Treating peritoneal carcinomatosis with curcumin and HIPEC has several advantages over standard regimen therapy. The combination of curcumin and HIPEC has the potential to reduce the formation of tissue adhesions,52,75,76 an important factor in recurrent disease. Finally, the use of HIPEC reduces the systemic toxicity associated with systemic delivery of chemotherapeutic agents, thereby reducing the side effects of treatment.
Significant limitations of treating peritoneal carcinomatosis with curcumin and HIPEC are cost and availability compared to standard therapeutics.9,77 At present, limited data exists supporting the efficacy of curcumin and HIPEC as a novel therapeutic. Finally, the procedure is only applicable in select cases and may not be applicable to all colorectal or peritoneal surface malignancy patients.78 While the pairing of curcumin and HIPEC is an intriguing prospect, more work will need to study mechanisms of cell death, long-term toxicity, and therapeutic effects in clinical trials. Many novel agents, such as immunotherapies,79–81 oncolytic viruses,82 and nanotechnology, have shown promise in vitro but must be further tested in an in vivo setting to determine their impact on patient outcomes. Only once rigorous clinical trials have been conducted, and we will have a more complete understanding of the efficacy and safety of combining novel therapeutics such as curcumin with HIPEC in colorectal cancer.
In conclusion, this study not only highlights the need for a reevaluation of MMC’s role in HIPEC but also suggests that personalized approaches based on genetic factors should be explored to improve treatment outcomes for PC in colorectal cancer patients.
Acknowledgments
We acknowledge the support and resources provided by Loma Linda University throughout the research process. The access to necessary facilities, equipment and data has been essential for the completion of this study. The abstract (#1451) of this paper was presented at the American Association for Cancer Research (AACR) Annual Meeting 2020 as a poster presentation. The poster’s abstract was published in ‘Proceedings’ AACR Annual Meeting 2020; April 27–28, 2020, and June 22–24, 2020; Volume 80, issue 16 supplement; Philadelphia, PA. Published abstract is available online: https://aacrjournals.org/cancerres/article/80/16_Supplement/1451/641352/Abstract-1451-Hyperthermic-conditions-improve.
Disclosure
No potential conflict of interest was reported by any of the authors.
References
1. Franko J, Shi Q, Meyers JP, et al. Prognosis of patients with peritoneal metastatic colorectal cancer given systemic therapy: an analysis of individual patient data from prospective randomised trials from the Analysis and Research in Cancers of the Digestive System (ARCAD) database. Lancet Oncol. 2016;17(12):1709–1719. doi:10.1016/S1470-2045(16)30500-9
2. Dawson LE, Russell AH, Tong D, Wisbeck WM. Adenocarcinoma of the sigmoid colon: sites of initial dissemination and clinical patterns of recurrence following surgery alone. J Surgical Oncol. 1983;22(2):95–99. doi:10.1002/jso.2930220208
3. Klaver YL, Lemmens VE, Creemers GJ, Rutten HJ, Nienhuijs SW, de Hingh IH. Population-based survival of patients with peritoneal carcinomatosis from colorectal origin in the era of increasing use of palliative chemotherapy. Ann Oncol. 2011;22(10):2250–2256. doi:10.1093/annonc/mdq762
4. Verwaal VJ, van Ruth S, de Bree E, et al. Randomized trial of cytoreduction and hyperthermic intraperitoneal chemotherapy versus systemic chemotherapy and palliative surgery in patients with peritoneal carcinomatosis of colorectal cancer. J Clin Oncol. 2003;21(20):3737–3743. doi:10.1200/JCO.2003.04.187
5. Sugarbaker PH, Alderman R, Edwards G, et al. Prospective morbidity and mortality assessment of cytoreductive surgery plus perioperative intraperitoneal chemotherapy to treat peritoneal dissemination of appendiceal mucinous malignancy. Ann Surg Oncol. 2006;13(5):635–644. doi:10.1245/ASO.2006.03.079
6. da Silva RG, Sugarbaker PH. Analysis of prognostic factors in seventy patients having a complete cytoreduction plus perioperative intraperitoneal chemotherapy for carcinomatosis from colorectal cancer. J Am Coll Surg. 2006;203(6):878–886. doi:10.1016/j.jamcollsurg.2006.08.024
7. Marz L, Piso P. Treatment of peritoneal metastases from colorectal cancer. Gastroenterol Rep. 2015;3(4):298–302. doi:10.1093/gastro/gov044
8. Bachmann C, Sautkin I, Nadiradze G, et al. Technology development of hyperthermic pressurized intraperitoneal aerosol chemotherapy (hPIPAC). Surg Endosc. 2021;35(11):6358–6365. doi:10.1007/s00464-021-08567-y
9. Weinreich J, Struller F, Sautkin I, et al. Chemosensitivity of various peritoneal cancer cell lines to HIPEC and PIPAC: comparison of an experimental duplex drug to standard drug regimens in vitro. Invest New Drugs. 2019;37(3):415–423. doi:10.1007/s10637-018-0641-6
10. Daniel SK, Sun BJ, Lee B. PIPAC for gastrointestinal malignancies. J Clin Med. 2023;12(21):1.
11. Schell F, Kefleyesus A, Benzerdjeb N, et al. Influence of extraperitoneal metastases on the curative-intent management of colorectal peritoneal metastases. Ann Surg Oncol. 2023;30(7):4444–4454. doi:10.1245/s10434-023-13279-9
12. Ornella MSC, Badrinath N, Kim KA, et al. Immunotherapy for peritoneal carcinomatosis: challenges and prospective outcomes. Cancers. 2023;15(8):2383. doi:10.3390/cancers15082383
13. Taliento C, Restaino S, Scutiero G, et al. Pressurized intraperitoneal aerosol chemotherapy (PIPAC) with cisplatin and doxorubicin in patients with ovarian cancer: a systematic review. Eur J Surg Oncol. 2023;49(12):107250. doi:10.1016/j.ejso.2023.107250
14. Fallows M, Samant A, Wilson H, Mirnezami R. A systematic review of surgical management strategies in the treatment of peritoneal carcinomatosis of neuroendocrine origin. Curr Oncol. 2023;30(7):6316–6329. doi:10.3390/curroncol30070466
15. Klaver CE, Musters GD, Bemelman WA, et al. Adjuvant hyperthermic intraperitoneal chemotherapy (HIPEC) in patients with colon cancer at high risk of peritoneal carcinomatosis; the COLOPEC randomized multicentre trial. BMC Cancer. 2015;15:428. doi:10.1186/s12885-015-1430-7
16. Sommariva A, Tonello M, Coccolini F, et al. Colorectal cancer with peritoneal metastases: the impact of the results of PROPHYLOCHIP, COLOPEC, and PRODIGE 7 trials on peritoneal disease management. Cancers. 2022;15(1):165. doi:10.3390/cancers15010165
17. Huang CQ, Min Y, Wang SY, et al. Cytoreductive surgery plus hyperthermic intraperitoneal chemotherapy improves survival for peritoneal carcinomatosis from colorectal cancer: a systematic review and meta-analysis of current evidence. Oncotarget. 2017;8(33):55657–55683. doi:10.18632/oncotarget.17497
18. Turaga K, Levine E, Barone R, et al. Consensus guidelines from The American Society of Peritoneal Surface Malignancies on standardizing the delivery of hyperthermic intraperitoneal chemotherapy (HIPEC) in colorectal cancer patients in the United States. Ann Surg Oncol. 2014;21(5):1501–1505. doi:10.1245/s10434-013-3061-z
19. Nadler A, McCart JA, Govindarajan A. Peritoneal carcinomatosis from colon cancer: a systematic review of the data for cytoreduction and intraperitoneal chemotherapy. Clin Colon Rectal Surg. 2015;28(4):234–246. doi:10.1055/s-0035-1564431
20. Murono K, Kawai K, Hata K, et al. Regimens of intraperitoneal chemotherapy for peritoneal carcinomatosis from colorectal cancer. Anticancer Res. 2018;38(1):15–22. doi:10.21873/anticanres.12186
21. Diaz Osterman CJ, Gonda A, Stiff T, et al. Curcumin induces pancreatic adenocarcinoma cell death via reduction of the inhibitors of apoptosis. Pancreas. 2016;45(1):101–109. doi:10.1097/MPA.0000000000000411
22. Shehzad A, Lee J, Lee YS. Curcumin in various cancers. Biofactors. 2013;39(1):56–68. doi:10.1002/biof.1068
23. Chainani-Wu N. Safety and anti-inflammatory activity of curcumin: a component of tumeric (Curcuma longa). J Altern Complement Med. 2003;9(1):161–168. doi:10.1089/107555303321223035
24. Zhang P, Lai ZL, Chen HF, et al. Curcumin synergizes with 5-fluorouracil by impairing AMPK/ULK1-dependent autophagy, AKT activity and enhancing apoptosis in colon cancer cells with tumor growth inhibition in xenograft mice. J Exp Clin Cancer Res. 2017;36(1):190. doi:10.1186/s13046-017-0661-7
25. Shakibaei M, Buhrmann C, Kraehe P, Shayan P, Lueders C, Goel A. Curcumin chemosensitizes 5-fluorouracil resistant MMR-deficient human colon cancer cells in high density cultures. PLoS One. 2014;9(1):e85397. doi:10.1371/journal.pone.0085397
26. Garcea G, Jones DJ, Singh R, et al. Detection of curcumin and its metabolites in hepatic tissue and portal blood of patients following oral administration. Br J Cancer. 2004;90(5):1011–1015. doi:10.1038/sj.bjc.6601623
27. Sharma RA, Euden SA, Platton SL, et al. Phase I clinical trial of oral curcumin: biomarkers of systemic activity and compliance. Clin Cancer Res. 2004;10(20):6847–6854. doi:10.1158/1078-0432.CCR-04-0744
28. Garcea G, Berry DP, Jones DJ, et al. Consumption of the putative chemopreventive agent curcumin by cancer patients: assessment of curcumin levels in the colorectum and their pharmacodynamic consequences. Cancer Epidemiol Biomarkers Prev. 2005;14(1):120–125. doi:10.1158/1055-9965.120.14.1
29. Carroll RE, Benya RV, Turgeon DK, et al. Phase IIa clinical trial of curcumin for the prevention of colorectal neoplasia. Cancer Prev Res. 2011;4(3):354–364. doi:10.1158/1940-6207.CAPR-10-0098
30. Zhang W, Cui T, Liu L, et al. Improving anti-tumor activity of curcumin by polymeric micelles in thermosensitive hydrogel system in colorectal peritoneal carcinomatosis model. J Biomed Nanotechnol. 2015;11(7):1173–1182. doi:10.1166/jbn.2015.2073
31. Xu XY, Meng X, Li S, Gan RY, Li Y, Li HB. Bioactivity, health benefits, and related molecular mechanisms of curcumin: current progress, challenges, and perspectives. Nutrients. 2018;10(10):1553. doi:10.3390/nu10101553
32. Khan S, Jutzy JM, Aspe JR, McGregor DW, Neidigh JW, Wall NR. Survivin is released from cancer cells via exosomes. Apoptosis. 2011;16(1):1–12. doi:10.1007/s10495-010-0534-4
33. de Cuba EM, Kwakman R, Knol DL, et al. Cytoreductive surgery and HIPEC for peritoneal metastases combined with curative treatment of colorectal liver metastases: systematic review of all literature and meta-analysis of observational studies. Cancer Treat Rev. 2013;39(4):321–327. doi:10.1016/j.ctrv.2012.11.003
34. Goodman MD, McPartland S, Detelich D, Saif MW. Chemotherapy for intraperitoneal use: a review of hyperthermic intraperitoneal chemotherapy and early post-operative intraperitoneal chemotherapy. J Gastrointest Oncol. 2016;7(1):45–57. doi:10.3978/j.issn.2078-6891.2015.111
35. Lemoine L, Sugarbaker P, Van der Speeten K. Drugs, doses, and durations of intraperitoneal chemotherapy: standardising HIPEC and EPIC for colorectal, appendiceal, gastric, ovarian peritoneal surface malignancies and peritoneal mesothelioma. Int J Hyperthermia. 2017;33(5):582–592. doi:10.1080/02656736.2017.1291999
36. Zhu C, Fang Z, Peng L, Gao F, Peng W, Song F. Curcumin suppresses the progression of colorectal cancer by improving immunogenic cell death caused by irinotecan. Chemotherapy. 2022;67(4):211–222. doi:10.1159/000518121
37. Ojo OA, Adeyemo TR, Rotimi D, et al. Anticancer properties of curcumin against colorectal cancer: a review. Front Oncol. 2022;12:881641. doi:10.3389/fonc.2022.881641
38. Li M, Guo T, Lin J, et al. Curcumin inhibits the invasion and metastasis of triple negative breast cancer via Hedgehog/Gli1 signaling pathway. J Ethnopharmacol. 2022;283:114689. doi:10.1016/j.jep.2021.114689
39. Firouzi Amandi A, Jokar E, Eslami M, et al. Enhanced anti-cancer effect of artemisinin- and curcumin-loaded niosomal nanoparticles against human colon cancer cells. Med Oncol. 2023;40(6):170. doi:10.1007/s12032-023-02032-7
40. He Q, Liu C, Wang X, et al. Exploring the mechanism of curcumin in the treatment of colon cancer based on network pharmacology and molecular docking. Front Pharmacol. 2023;14:1102581. doi:10.3389/fphar.2023.1102581
41. Shojaei M, Sahebkar A, Khorvash F, Fallahpour S, Askari G, Bagherniya M. The effects of phytosomal curcumin supplementation on clinical symptoms, and inflammatory and oxidative stress biomarkers in patients with migraine: a protocol for a randomized double-blind placebo-controlled trial. Avicenna J Phytomed. 2023;13(1):45–57. doi:10.22038/AJP.2022.21242
42. Hu Z, Tang Y, Jiang B, Xu Y, Liu S, Huang C. Functional liposome loaded curcumin for the treatment of Streptococcus mutans biofilm. Front Chem. 2023;11:1160521. doi:10.3389/fchem.2023.1160521
43. Garcia-Cuellar CM, Hernandez-Delgadillo R, Torres-Betancourt JA, et al. Cumulative antitumor effect of bismuth lipophilic nanoparticles and cetylpyridinium chloride in inhibiting the growth of lung cancer. J Appl Biomater Funct Mater. 2023;21:22808000231161177. doi:10.1177/22808000231161177
44. Li M, Tao J, Qian R, et al. Development of alternative herbals remedy for gastric cancer based on transcriptomic analysis of immune infiltration and ferroptosis. Front Genet. 2023;14:1086368. doi:10.3389/fgene.2023.1086368
45. Idoudi S, Bedhiafi T, Hijji YM, Billa N. Curcumin and derivatives in nanoformulations with therapeutic potential on colorectal cancer. AAPS Pharm Sci Tech. 2022;23(5):115. doi:10.1208/s12249-022-02268-y
46. Weng W, Goel A. Curcumin and colorectal cancer: an update and current perspective on this natural medicine. Semin Cancer Biol. 2022;80:73–86. doi:10.1016/j.semcancer.2020.02.011
47. Wang Y, Lu J, Jiang B, Guo J. The roles of curcumin in regulating the tumor immunosuppressive microenvironment. Oncol Lett. 2020;19(4):3059–3070. doi:10.3892/ol.2020.11437
48. Irving GR, Iwuji CO, Morgan B, et al. Combining curcumin (C3-complex, Sabinsa) with standard care FOLFOX chemotherapy in patients with inoperable colorectal cancer (CUFOX): study protocol for a randomised control trial. Trials. 2015;16(1):110. doi:10.1186/s13063-015-0641-1
49. Sinha D, Biswas J, Sung B, Aggarwal BB, Bishayee A. Chemopreventive and chemotherapeutic potential of curcumin in breast cancer. Curr Drug Targets. 2012;13(14):1799–1819. doi:10.2174/138945012804545632
50. Sadeghi M, Dehnavi S, Asadirad A, et al. Curcumin and chemokines: mechanism of action and therapeutic potential in inflammatory diseases. Inflammopharmacology. 2023;31(3):1069–1093. doi:10.1007/s10787-023-01136-w
51. Zhang H, Li H, Wang H, Lei S, Yan L. Overexpression of TRPM7 promotes the therapeutic effect of curcumin in wound healing through the STAT3/SMAD3 signaling pathway in human fibroblasts. Burns. 2023;49(4):889–900. doi:10.1016/j.burns.2022.06.016
52. Lev-Ari S, Strier L, Kazanov D, et al. Curcumin synergistically potentiates the growth-inhibitory and pro-apoptotic effects of celecoxib in osteoarthritis synovial adherent cells. Rheumatology. 2006;45(2):171–177. doi:10.1093/rheumatology/kei132
53. Naylor G, Julian L, Watson-Bryce S, Mullin M, Nibbs RJ, Olson MF. Immunogenic death of hepatocellular carcinoma cells in mice expressing caspase-resistant ROCK1 is not replicated by ROCK inhibitors. Cancers. 2022;14(23). doi:10.3390/cancers14235943
54. Bao X, Xie L. Targeting purinergic pathway to enhance radiotherapy-induced immunogenic cancer cell death. J Exp Clin Cancer Res. 2022;41(1):222. doi:10.1186/s13046-022-02430-1
55. Zhou Y, Liu C, Song H. Innate immunomodulatory nanodevices for cancer therapy: a review. J Biomed Nanotechnol. 2022;18(2):293–318. doi:10.1166/jbn.2022.3241
56. Mao L, Zhou JJ, Xiao Y, et al. Immunogenic hypofractionated radiotherapy sensitising head and neck squamous cell carcinoma to anti-PD-L1 therapy in MDSC-dependent manner. Br J Cancer. 2023;128(11):2126–2139. doi:10.1038/s41416-023-02230-0
57. Calvillo-Rodriguez KM, Lorenzo-Anota HY, Rodriguez-Padilla C, Martinez-Torres AC, Scott-Algara D. Immunotherapies inducing immunogenic cell death in cancer: insight of the innate immune system. Front Immunol. 2023;14:1294434. doi:10.3389/fimmu.2023.1294434
58. Aarts F, Hendriks T, Boerman OC, Koppe MJ, Oyen WJ, Bleichrodt RP. A comparison between radioimmunotherapy and hyperthermic intraperitoneal chemotherapy for the treatment of peritoneal carcinomatosis of colonic origin in rats. Ann Surg Oncol. 2007;14(11):3274–3282. doi:10.1245/s10434-007-9509-2
59. Gajardo JA, Matute J, Charles R, et al. Starting a peritoneal carcinomatosis treatment program in a developing country: a prospective analysis. J Surg Oncol. 2021;124(7):1154–1160. doi:10.1002/jso.26626
60. Lehmann K, Rickenbacher A, Jang JH, et al. New insight into hyperthermic intraperitoneal chemotherapy: induction of oxidative stress dramatically enhanced tumor killing in in vitro and in vivo models. Ann Surg. 2012;256(5):730–738. doi:10.1097/SLA.0b013e3182737517
61. Woeste MR, Philips P, Egger ME, Scoggins CR, McMasters KM, Martin RCG. Optimal perfusion chemotherapy: a prospective comparison of mitomycin C and oxaliplatin for hyperthermic intraperitoneal chemotherapy in metastatic colon cancer. J Surg Oncol. 2020;121(8):1298–1305. doi:10.1002/jso.25920
62. Choudhary HB, Mandlik SK, Mandlik DS. Role of p53 suppression in the pathogenesis of hepatocellular carcinoma. World J Gastrointest Pathophysiol. 2023;14(3):46–70. doi:10.4291/wjgp.v14.i3.46
63. Ghantous L, Volman Y, Hefez R, et al. The DNA damage response pathway regulates the expression of the immune checkpoint CD47. Commun Biol. 2023;6(1):245. doi:10.1038/s42003-023-04615-6
64. Gao SB, Li KL, Qiu H, et al. Enhancing chemotherapy sensitivity by targeting PcG via the ATM/p53 pathway. Am J Cancer Res. 2017;7(9):1874–1883.
65. Ottaggio L, Campomenosi P, Fronza G, et al. Stable formation of mutated p53 multimers in a Chinese hamster cell line causes defective p53 nuclear localization and abrogates its residual function. J Cell Biochem. 2006;98(6):1689–1700. doi:10.1002/jcb.20921
66. Zhang J, Li L, Yin J, Zhang X, Zheng Y, Feng R. Study on the thermal stability of nab-paclitaxel during hyperthermic intraperitoneal chemotherapy. BMC Pharmacol Toxicol. 2023;24(1):13. doi:10.1186/s40360-023-00653-2
67. Murata S, Yamamoto H, Naitoh H, et al. Feasibility and safety of hyperthermic intraperitoneal chemotherapy using 5-fluorouracil combined with cisplatin and mitomycin C in patients undergoing gastrectomy for advanced gastric cancer. J Surg Oncol. 2017;116(8):1159–1165. doi:10.1002/jso.24771
68. Spiliotis J, Rogdakis A, Vaxevanidou A, Datsis A, Zacharis G, Christopoulou A. Morbidity and mortality of cytoreductive surgery and hyperthermic intraperitoneal chemotherapy in the management of peritoneal carcinomatosis. J BUON. 2009;14(2):259–264.
69. Fan B, Bu Z, Zhang J, et al. Phase II trial of prophylactic hyperthermic intraperiton eal chemotherapy in patients with locally advanced gastric cancer after curative surgery. BMC Cancer. 2021;21(1):216. doi:10.1186/s12885-021-07925-2
70. Kusamura S, Baratti D, Younan R, et al. Impact of cytoreductive surgery and hyperthermic intraperitoneal chemotherapy on systemic toxicity. Ann Surg Oncol. 2007;14(9):2550–2558. doi:10.1245/s10434-007-9429-1
71. Ceelen WP, Peeters M, Houtmeyers P, Breusegem C, De Somer F, Pattyn P. Safety and efficacy of hyperthermic intraperitoneal chemoperfusion with high-dose oxaliplatin in patients with peritoneal carcinomatosis. Ann Surg Oncol. 2008;15(2):535–541. doi:10.1245/s10434-007-9648-5
72. Sugarbaker PH, Stuart OA, Carmignani CP. Pharmacokinetic changes induced by the volume of chemotherapy solution in patients treated with hyperthermic intraperitoneal mitomycin C. Cancer Chemother Pharmacol. 2006;57(5):703–708. doi:10.1007/s00280-005-0074-8
73. Jacquet P, Averbach A, Stephens AD, Stuart OA, Chang D, Sugarbaker PH. Heated intraoperative intraperitoneal mitomycin C and early postoperative intraperitoneal 5-fluorouracil: pharmacokinetic studies. Oncology. 1998;55(2):130–138. doi:10.1159/000011847
74. Fujimoto S, Takahashi M, Kobayashi K, et al. Metabolic changes in cimetidine treatment for scald injury on the peritoneo-serosal surface in far-advanced gastric cancer patients treated by intraperitoneal hyperthermic perfusion. Surg Today. 1993;23(5):396–401. doi:10.1007/BF00309496
75. Fan Z, Jing H, Yao J, et al. The protective effects of curcumin on experimental acute liver lesion induced by intestinal ischemia-reperfusion through inhibiting the pathway of NF-kappaB in a rat model. Oxid Med Cell Longev. 2014;2014:191624. doi:10.1155/2014/191624
76. Chen MJ, Cheng YM, Lai PH, Wu JF, Hsu YC. In vitro biocompatibility of thermally gelling liquid mucoadhesive loaded curcuminoids in colorectal cancer chemoprevention. Int J Colorectal Dis. 2012;27(7):869–878. doi:10.1007/s00384-011-1393-3
77. Spiliotis J, Tentes AA, Vaxevanidou A, et al. Cytoreductive surgery and hyperthermic intraperitoneal chemotherapy in the management of peritoneal carcinomatosis. Preliminary results and cost from two centers in Greece. J BUON. 2008;13(2):205–210.
78. Canda AE, Arslan C, Terzi C, et al. Treatment of intraoperatively detected peritoneal carcinomatosis of colorectal origin with cytoreductive surgery and intraperitoneal chemotherapy. World J Surg Oncol. 2018;16(1):70.
79. Aarts F, Bleichrodt RP, de Man B, Lomme R, Boerman OC, Hendriks T. The effects of adjuvant experimental radioimmunotherapy and hyperthermic intraperitoneal chemotherapy on intestinal and abdominal healing after cytoreductive surgery for peritoneal carcinomatosis in the rat. Ann Surg Oncol. 2008;15(11):3299–3307. doi:10.1245/s10434-008-0070-4
80. Luo C, Cen S, Ding G, Wu W. Mucinous colorectal adenocarcinoma: clinical pathology and treatment options. Cancer Commun. 2019;39(1):13. doi:10.1186/s40880-019-0361-0
81. Peng HH, Huang YT, Cheng ZX, Lee CL, Lin CT. Immunomodulating therapy by picibanil-based imiquimod and virotherapy for advanced uterine cancer after laparoscopic surgery. Gynecol Minim Invasive Ther. 2021;10(3):191–194. doi:10.4103/GMIT.GMIT_153_20
82. Bienkowska A, Kuzmicka W, Ciepiela O, Ochocki J, Malecki M. Increased temperature facilitates adeno-associated virus vector transduction of colorectal cancer cell lines in a manner dependent on heat shock protein signature. Biomed Res Int. 2020;2020:9107140. doi:10.1155/2020/9107140
© 2024 The Author(s). This work is published and licensed by Dove Medical Press Limited. The full terms of this license are available at https://www.dovepress.com/terms.php and incorporate the Creative Commons Attribution - Non Commercial (unported, v3.0) License.
By accessing the work you hereby accept the Terms. Non-commercial uses of the work are permitted without any further permission from Dove Medical Press Limited, provided the work is properly attributed. For permission for commercial use of this work, please see paragraphs 4.2 and 5 of our Terms.