Back to Journals » Nanotechnology, Science and Applications » Volume 17
The Chemical Modification to Improve Solubility of Chitosan and Its Derivatives Application, Preparation Method, Toxicity as a Nanoparticles
Authors Suryani S , Chaerunisaa AY, Joni IM, Ruslin R , Aspadiah V, Anton A, Sartinah A, Ramadhan LOAN
Received 1 December 2023
Accepted for publication 21 February 2024
Published 7 March 2024 Volume 2024:17 Pages 41—57
DOI https://doi.org/10.2147/NSA.S450026
Checked for plagiarism Yes
Review by Single anonymous peer review
Peer reviewer comments 2
Editor who approved publication: Professor Kattesh Katti
Suryani Suryani,1,2 Anis Yohana Chaerunisaa,3,4 I Made Joni,5,6 Ruslin Ruslin,2 Vica Aspadiah,2 Anton Anton,7 Ari Sartinah,2 La Ode Ahmad Nur Ramadhan8
1Doctor of Pharmacy Study Program, Faculty of Pharmacy, Padjadjaran University, Sumedang, Indonesia; 2Department of Pharmacy, Faculty of Pharmacy, Halu Oleo University, Kendari, Indonesia; 3Department of Pharmaceutics and Pharmaceutical Technology, Faculty of Pharmacy, Padjadjaran University, Sumedang, Indonesia; 4Dosage Form Development Research Centre, Faculty of Pharmacy, Padjadjaran University, Sumedang, Indonesia; 5Department of Physics, Faculty of Mathematics and Natural Sciences, Padjadjaran University, Sumedang, Indonesia; 6Functional Nano Powder University Centre of Excellence, Padjadjaran University, Sumedang, Indonesia; 7Department of Biology, Faculty of Mathematics and Natural Sciences, Halu Oleo University, Kendari, Indonesia; 8Department of Chemistry, Faculty of Mathematics and Natural Sciences, Halu Oleo University, Kendari, Indonesia
Correspondence: Anis Yohana Chaerunisaa; Suryani Suryani, Email [email protected]; [email protected]
Abstract: Chitosan is a functional polymer in the pharmaceutical field, including for nanoparticle drug delivery systems. Chitosan-based nanoparticles are a promising carrier for a wide range of therapeutic agents and can be administered in various routes. Solubility is the main problem for its production and utilization in large-scale industries. Chitosan modifications have been employed to enhance its solubility, including chemical modification. Many reviews have reported the chemical modification but have not focused on the specific characteristics obtained. This review focused on the modification to improve chitosan solubility. Additionally, this review also focused on the application of chitosan derivatives in nanoparticle drug delivery systems since very few similar reviews have been reported. The specific method for chitosan derivative-based nanoparticles was also reported and the latest report of chitosan, chitosan derivative, and chitosan toxicity were also described.
Keywords: polymer, chitosan derivatives, solubility enhancement, drug delivery system
Graphical Abstract:
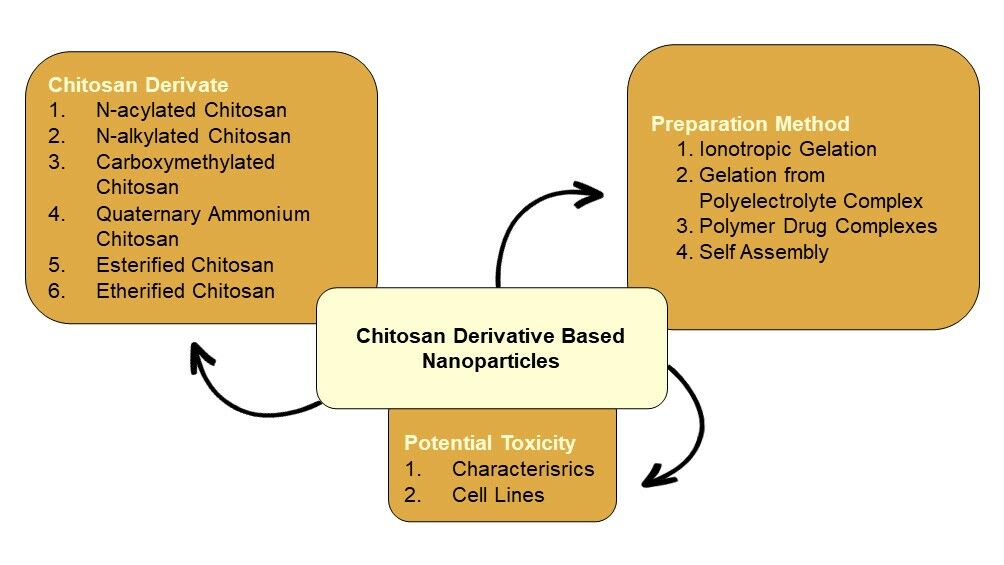
Introduction
Chitosan, a deacetylated product of chitin, is a functional polymer in the pharmaceutical field due to its biodegradability, biocompatibility, bioadhesive, and non-toxic properties. Chitosan has been employed in nanoparticle drug delivery systems. Various drugs can be loaded into chitosan-based nanoparticles including drugs with small molecules, polynucleotides, and proteins.1 Chitosan-based nanoparticles for the controlled release of anticancer agents have been reported.2 The small size of the nanoparticle can increase the drug’s capacity to penetrate cells passively or actively.3 Chitosan-mediated membrane tight junction disruption increases the infusion of drugs across the cell.4 Chitosan nanoparticles are a promising carrier for oral delivery macromolecules.5 Various drug administration routes using chitosan nanoparticles as a material have been reported, including oral, pulmonary, transdermal, ocular, and nasal routes.1,2,4 Application of chitosan in nanoparticle drug delivery systems is limited because of its insolubility at neutral pH. Chitosan is only soluble in acid solution which is not conducive for some drugs.6 Solubility is the biggest limitation of chitosan for the development process and its utilization in the large-scale industry. For nanoparticle production, most of the nanoparticles prepared by using insoluble polymer involve organic solvent, heat, and high shear force can lead to the degradation of active substance, otherwise the nanoparticles prepared using soluble polymer offer simple preparation without organic solvent, heat, and high shear force.7 Furthermore, at pH ≥6, chitosan will precipitate and form aggregation. Therefore, when administered under neutral or basic physiological pH will form precipitation and lead to adverse effects.8 Chitosan modifications have been employed to improve its physicochemical and biological properties, including chemical modification of chitosan. Its reactive amino and hydroxyl groups allow for potential modifications using grafting techniques and ionic interactions.2,9 Many articles have reported the chitosan modification but do not focus on the specific target characteristics obtained. In this review, the author focuses on the chitosan modification to improve its solubility. The modification employed to enhance chitosan solubility, includes acylation, alkylation, carboxylation, quaternization, esterification, and etherification. This report also reviews the application of chitosan derivatives in nanoparticle production, while very few reviews have been reported before. This review also reports the specific method used for chitosan derivative-based nanoparticle preparation and the toxicity of chitosan, chitosan derivatives, and chitosan nanoparticles. Figure 1 displays the structures of chitin and chitosan.
![]() |
Figure 1 The chemical structure of chitin and chitosan. |
Solubility Improvement of Chitosan
Acylated Chitosan
Acylation of chitosan destroys the hydrogen bonding of chitosan and improves the solubility of chitosan.10 Amine group of chitosan is more reactive than the primary hydroxyl group; therefore, the acylation reaction is mostly in the amine group.11 Acylation of chitosan is performed by introducing aromatic acyl or aliphatic group.12 The synthesized reaction of N-acylated chitosan is shown in Figure 2.
![]() |
Figure 2 The synthetized reaction of N-acylated chitosan. |
N-acylated chitosan was synthesized by introducing anhydrides to chitosan such as succinic, propionic, lauric, butyric, stearic, itaconic, and myristic anhydrides. Short-chain acylated chitosan shows higher solubility than long-chain acylated chitosan.13 Introduction of various cyclic acid anhydrides to chitosan could improve the water solubility of chitosan in various pH; the initial chitosan only dissolves in pH below 6.5, while the derivatives show a solubility of pH above 7–8.14 Hirano et al prepared N-Fatty acyl chitosan, which is water soluble, by treatment with various anhydrides.15
N-acylation of chitosan has been applied to nanoparticle delivery systems. It has been reported that N-acylated chitosan is functionalized with gold nanoparticles for gene therapy. The transfection test showed that the percentage of transfection of N-acylated chitosan-based nanoparticles was higher compared to chitosan.16 Cho et al showed the application of N-acylated chitosan nanoparticles as a protein carrier.17 N-acylated chitosan nanoparticles were applied for the controlled release of Vitamin C. The study showed that controlled release properties of vitamin C at pH 1.3 and pH 7.4.18 N-acetylated chitosan nanoparticles have been employed as a carrier for thymoquinone for kidney protection after cyclophosphamide chemotherapy. The study showed that the thymoquinone-N-alkylated chitosan nanoparticles were effectively delivered to the kidney and improved its protective efficacy against cyclophosphamide-induced hemorrhagic cystitis.19 N-acylated chitosan was used for the stabilization of glabridin, a polyphenolic compound isolated from licorice root by forming a chitosan nano-complex. It has been shown that chitosan derivatives can be used as a stabilizer for polyphenolic compounds and potentially applied to skin-whitening agents.20 N-acylated chitosan interacts with liposomes effectively, forming Chito-liposome.21 The important characteristics of nanoparticles-based acylated chitosan are shown in Table 1.
![]() |
Table 1 The Important Characteristics of Nanoparticle-Based Acylated Chitosan |
Alkylated Chitosan
The reaction using halogenated alkanes and Schiff’s base are two common methods used to synthesize N-alkylated chitosan.22 The reaction using halogenated alkanes is performed by introducing an alkyl to chitosan. The solubility depends on the length of the alkyl group introduced to chitosan; the lower the length of the alkyl group, the higher the solubility of alkylated chitosan.10 Reduction of hydrogen bonding between molecules of chitosan occurs because of the presence of the alkyl group, thereby increasing water solubility.12 The synthesized reaction of N-alkylated chitosan is shown in Figure 3.
![]() |
Figure 3 The synthetized reaction of N-alkylated chitosan. |
The Schiff’s base was performed by the Schiff’s base reaction with an aldehyde and the reduction of NaBH4, resulting in N-alkylated chitosan. The Schiff’s base reaction with methyl iodide results in the quaternization of chitosan which improves water solubility.23 N-alkylated chitosan was also synthesized using Michael’s addition reaction of hydroxyethyl acrylate and chitosan to improve the water solubility of chitosan.24 The introduction of disaccharides to chitosan produces water-soluble N-alkylated chitosan.25 Palacio et al found that N-alkylated chitosan by methyl groups improves water solubility due to the positive charge caused by the quaternary ammonium group and the derivatives are soluble in a wide range of pH from 3 to 14, whereas non-derivative chitosan is only soluble in acid pH.26 O-alkylated chitosan was studied by Chen et al who stated that the alkylated chitosan was designed using a bonding agent (N, N’-carbonyldiimidazole) in an ionic liquid solvent. The solubility test of O-alkylated chitosan showed solubility improvement in aqueous acetic acid solution (w/w; 0.1%).27
The application of alkylated chitosan in nano drug delivery systems was reported by Robles et al. The alkylated chitosan was employed for insulin nanoparticle preparation, and it showed that the insulin loading capacity was higher compared to that of unmodified chitosan. The release of insulin from nanoparticles was slower, and it has potential for sustained release application.28 The O-alkyl chitosan was used to prepare an amphiphilic N, N, N-trimethyl-O-alkyl chitosan derivative (TMAC). TMAC was used to prepare the self-assembled nanoparticle for peptide nucleic acid (PNA) cellular delivery. The study showed that the cellular uptake of PNA was higher than non-TMAC-based nanoparticles.29 The N-N-alkyl chitosan was used to prepare nanocomposite films containing silver nanoparticles for antimicrobial activity.30 The important characteristics of nanoparticles-based alkylated chitosan are shown in Table 2.
![]() |
Table 2 The Important Characteristics of Nanoparticle-Based Alkylated Chitosan |
Carboxylated Chitosan
Carboxylated chitosan is water soluble in neutral and alkaline pH. Carboxylated chitosan is obtained by introducing carboxylic acid (glyoxylic or chloroalkanoic acid) to the C6-OH group or C2-NH2 group of chitosan.10 Carboxymethylation is the most common reaction to obtain the carboxylated chitosan. The solubility of carboxylated chitosan in water depends on the degree of the carboxymethylation of chitosan.12 The types of the obtained carboxymethyl chitosan (CMCS) can be N-CMCS, O-CMCS, N, O-CMCS and N, N-CMCS.31,32 The reaction of carboxymethylation is shown in Figure 4. N-carboxymethyl chitosan was prepared using glyoxylic acid and sodium cyanoborohydride.32 Song et al also prepared N-carboxymethyl chitosan using chloroacetic acid in water (neutral pH), and Na2CO3 was used to control the reaction system at neutral pH of 7.33 The O-carboxymethyl chitosan was described by Mohamed et al using monochloroacetic acid.34 The preparation of O, N-carboxymethyl chitosan was reported by Patale et al. The O, N-carboxymethyl chitosan was prepared by adding isopropyl alcohol to chitosan. To the resulting slurry, sodium hydroxy solution was then added and stirred for 45 minutes. The solid monochloroacetic acid was then added to the alkaline slurry and stirred over 20 minutes.35 The preparation of hydrazide-based O-CMCS Schiff’s bases that are soluble in water through in situ reaction was reported by Manmohan et al.36 The reaction of carboxymethylation is shown in Figure 4.
![]() |
Figure 4 The synthetized reaction of carboxymethylation chitosan. |
Several studies have explored the various applications of carboxymethyl chitosan in nanoparticle development. For instance, carboxymethyl chitosan was utilized in the preparation of carboxymethyl chitosan-ZnO nanoparticles to exhibit antibacterial activity against S. aureus and E. coli.37 Additionally, O-carboxymethyl chitosan was employed in fabricating O-carboxymethyl chitosan/Fucoidan (O-CMCS-F) nanoparticles for the delivery of curcumin. This study demonstrated that these nanoparticles enhanced the cellular uptake of curcumin, suggesting their potential application in oral delivery systems and controlled release of the compound.38 In another study, carboxymethyl chitosan nanoparticles were employed for the intranasal delivery of carbamazepine, an antiepileptic drug, aiming to enhance drug delivery to the brain. The findings suggested that these nanoparticles have promise for nasal formulation.39 Feng et al illustrated the use of chitosan/O-carboxymethyl chitosan in creating doxorubicin hydrochloride nanoparticles for anticancer drug delivery. Their research indicated that CS/CMCS nanoparticles were both safe and effective for doxorubicin hydrochloride delivery.40 Mahjub et al reported the preparation of nanoparticles composed of N-trimethyl-O-carboxymethyl chitosan for oral delivery of enoxaparin, showcasing the potential of TMCMC in this application.41 Ultrasound-assisted techniques were utilized in another study to create carboxymethyl chitosan-based nanoparticles for the delivery of clindamycin HCL, demonstrating the effectiveness of this method in controlling the drug release of clindamycin.42 Xu et al presented a nanoparticle preparation method employing negatively charged CMCS and positively charged chitosan quaternary ammonium salt for an immunostimulatory effect. Their study suggested that HACC nanoparticles had potential as an immunological adjuvant.43 Table 3 outlines the significant characteristics of nanoparticles based on carboxylated chitosan.
![]() |
Table 3 The Important Characteristics of Nanoparticle-Based Carboxylated Chitosan |
Quaternization
The process of quaternization is a common method used to produce quaternary ammonium chitosan. This reaction typically occurs in the amine groups present in chitosan.12 There are three primary methods for quaternization: direct quaternary ammonium substitution, the open-loop reaction of epoxy derivatives, and N-alkylation.10 The enhanced solubility of quaternary ammonium chitosan is attributed to its positive charge.44 The initial synthesis of N, N, N trimethyl chitosan iodide (TMC), a derivative of quaternary ammonium chitosan, was conducted using formaldehyde, sodium borohydride, and CH3I. Dormard introduced a method known as the Dormard method, involving N-methyl-2-pyrrolidone, NaOH, and CH3I, to synthesize quaternary ammonium chitosan derivatives.45 Copolymers were obtained by grafting dodecyl aldehyde (DDA) and 5-bromomethyl trimethylammonium bromide (BPTA) onto chitosan. The resulting amphiphilic chitosan derivative displayed self-aggregation ability, potentially useful for drug delivery systems.44 Fan et al synthesized quaternary ammonium chitosan using (N-(3-chloro-2-hydroxypropyl) trimethyl ammonium chloride) and sodium hydroxide.46 Sajomsang et al successfully produced water-soluble 9-quaternary ammonium chitosan containing mono/disaccharides using a quaternizing agent called N-(3-chloro-2-hydroxypropyl) trimethylammonium chloride.47 The process of synthesizing quaternary ammonium chitosan is shown in Figure 5.
![]() |
Figure 5 The synthetized reaction of quaternary ammonium chitosan. |
Trimethyl chitosan (TMC) was used to develop nanoparticles loaded with insulin using poly (ɣ-glutamic acid) as a complexing agent.48,49 The hydro-soluble chitosan derivative, the N-(2-hydroxy) propyl 3 trimethyl ammonium chitosan chloride (HCTT), was used to obtain ribavirin-loaded nanoparticles. The study showed that the HCTT is promising for a controlled release of hydrophilic drugs.50 The preparation of HCTT nanoparticles loaded with albumin was also reported.51 TMC was also used to develop TMC-liposome-doxycycline nanoparticles. The nanoparticles showed superb inhibition of bacteria and biofilm and could potentially be used for periodontal treatment.52 Huang et al reported the formation of quaternary ammonium chitosan-fucoidan nanoparticles loaded with epigallocatechin gallate (EGCG) for enhancing the permeability and antibacterial activity of EGCG. The study suggested that the nanoparticles increased the bacterial membrane permeability, thus increasing the antibacterial activity of EGCG.53 The important characteristics of nanoparticles-based quartered chitosan are shown in Table 4.
![]() |
Table 4 The Important Characteristics of Nanoparticle-Based Quaternized Chitosan |
Chitosan Esterification
Chitosan esterification involves the reaction of chitosan with carboxylic acid or its derivatives.10 Wang et al documented the synthesis of water-soluble chitosan ester (p-aminobenzoyl chitosan ester) using Schiff’s base method.54 Another reported method involved creating sulfated chitosan by treating chitosan with a DMF-dichloroacetic acid mixture and chlorosulfonic acid.55 Chitosan acetate was obtained by varying the ratio of acetic acid to chitosan, while chitosan glutamate was prepared by dissolving chitosan in glutamic acid.56,57 In a patent by Yoon et al, the esterification process of chitosan was detailed using various carboxylic acids (acetic acid, formic acid, propionic acid, butyric acid, and valeric acid) along with trifluoroacetic anhydride (TFAA).11,58 The schematic representation of the esterification reaction of chitosan is illustrated in Figure 6.
![]() |
Figure 6 The synthetized reaction of esterification chitosan. |
The application of chitosan ester in nanoparticle drug delivery systems was reported by Bhattari et al. They reported the preparation of bovine serum protein loaded by chitosan lactate nanoparticle and the resulting nanoparticles were 10 nm in diameter. They also found that the encapsulation was 92% and exhibited a sustained release pattern.59 The study also found that CD73-specific siRNA-loaded chitosan lactate nanoparticles have an antitumor effect.60 Bovine serum albumin-loaded chitosan salt (lactate, aspartate, glutamate, and glycolate) nanoparticles were prepared using the ionic gelation method and the characteristics of the nanoparticles depended on the types of salt.57 Colonna et al presented the development of chitosan glutamate nanoparticles for prolidase enzyme delivery to treat autosomal disorders.61 The sulfated chitosan-amphotericin B nanoparticles were developed for treating Candida glabrata infections.62 The important characteristics of nanoparticles-based esterified chitosan are shown in Table 5.
![]() |
Table 5 The Important Characteristics of Nanoparticle-Based Esterified Chitosan |
Chitosan Etherification
A chitosan-etherified derivative is obtained by reacting the alkylating agent with chitosan.63 A chitosan-etherified derivative is water soluble and has good water retention, is nontoxic, bacteriostatic, and has wider applications in the pharmaceutical field.10,64 The methyl chitosan was prepared by reacting dimethyl sulfate and chitosan in an aqueous alkali/urea solution.65 N, O-carboxymethyl chitosan was derivatized from chitosan by adding NaOH and monochloroacetic acid. The etherification process was conducted in temperatures of 40–70°C.66 Cationic chitosan derivatives with long-chain alkyl (HDCC) were synthesized by using 2 kinds of etherifying agents, which are (2,3-epoxypropoxy) dodecyl dimethyl ammonium chloride and glycidyl trimethylammonium chloride.67 Yang et al found that the commonly used etherifying agents to produce etherified chitosan are 3-chloro-2-hydroxypropyl trimethylammonium chloride (CTA), 2.3,-epoxypropyl trimethyl ammonium chloride (ETA), and carboxyethyl groups.68 Hydroxy butyl chitosan (HBC) is water-soluble etherified chitosan, which is produced via etherification by reacting hydroxy butyl group and chitosan.69 The hydroxy-propyl chitosan was prepared by reacting chitosan and propylene epoxide under alkali conditions. The etherified chitosan obtained has good solubility in water.70 The following (Figure 7) is a figure of the interaction of chitosan (Black Line) with epoxide (Red Image) which can produce an ether and secondary amine (Black and Red Line).
![]() |
Figure 7 The synthetized reaction of etherification chitosan. chitosan (black line) and epoxide (red line. |
The application of etherified chitosan in nanoparticle drug delivery systems has garnered substantial attention due to its characteristics such as water solubility, biodegradability, and biocompatibility. Hydroxy butyl chitosan application in nanoparticle drug delivery system was reported by Sun et al, in which cetirizine-loaded deoxycholate-hydroxy butyl chitosan nanoparticles (CTZ: CDHBCs-NP) were synthesized as nasal nano vehicles to treat allergic rhinitis. The CTZ: CDHBCs-NP possesses high loading capacity, and drug release behavior might be potential as an anti-allergic nasal nano vehicle.71 Lu et al synthetized hydroxy-propyl chitosan nanoparticles using the ionic gelation method. The obtained nanoparticles were 40 nm in diameter, and the zeta potential was +47.7 mV.72 The important characteristics of nanoparticles-based etherified chitosan are shown in Table 6.
![]() |
Table 6 The Important Characteristics of Nanoparticle-Based Etherified Chitosan |
Chitosan Derivatives Nanoparticle Preparation
The process of preparing chitosan derivative nanoparticles commonly employs multiple techniques, including ionotropic gelation, gelation from Polyelectrolyte Complex (PEC) formation, Polymer-Drug Complexes, and self-assembly.
Ionotropic Gelation
The ionotropic gelation technique was initially introduced by Calvo et al in 1997. This method is capable of producing nanoparticles through electrostatic interactions between oppositely charged polymers under specific mechanical stirring conditions.73 Nanoparticles are defined as materials with sizes typically ranging from 1 to 100 nm. However, the nanoparticles generated using the ionic gelation method typically exhibit sizes larger than 100 nm.74 Several quaternized chitosans with stable, pH-independent positive charges have been used through this method, with the most well-known being N-trimethyl chitosan (TMC).
Gonzalez et al conducted the preparation of gold nanoparticles with chitosan, N-acylated chitosan, and chitosan oligosaccharide as DNA carriers. Chitosan (low molecular weight) acylation was performed by following the method reported by Remant Bahadur et al with slight modifications. The colloidal solution demonstrated excellent stability, which indicates the practical application of chitosan as a stabilizer. Additionally, chitosan oligosaccharide served as both a reducing agent and stabilizer, while the amino groups in chitosan enhanced its affinity with plasmid DNA by introducing positive charges. The size distributions of the produced gold nanoparticles ranged from 3 to 15 nm.75
However, while many nanoparticle synthesis methods yield a narrow particle size distribution, the ionotropic gelation method may not consistently achieve this. The polydispersity index (PDI) often exceeds 0.1, sometimes reaching up to 0.5. Consequently, the heterogeneity in particle size not only affects drug loading but can also restrict the interaction of particles with biological structures. Additionally, maintaining a constant speed in the dropping solution and controlled stirring are essential requirements for this method.76
Another study by Sayin et al developed Mono-N-carboxymethyl chitosan (MCC) and N-trimethyl chitosan (TMC) nanoparticles for non-invasive vaccine delivery. The nanoparticulate systems were prepared by using differently charged chitosan derivatives, N-trimethyl chitosan (TMC, polycationic), and mono-N-carboxymethyl chitosan (MCC, polyampholytic) for mucosal immunization. Nanoparticles were prepared using the ionic gelation method and loaded with tetanus toxoid (TT). Nanoparticles with high loading efficacy (>90% m/m) and particle size within the range of 40–400 nm with a negative surface charge for MCC and positive surface charge for TMC and chitosan were obtained. Both dispersion and nanoparticle systems formulated with chitosan derivatives were discovered to augment mucosal immune responses. The findings highlighted that the surface charge and particle size of carriers produced with chitosan play a crucial role in achieving an improved immune response. MCC elicited comparatively diminished immunological responses for TT in comparison to TMC and chitosan, however, it yielded the smallest nanoparticles with a significantly narrower size distribution and a high loading capacity. MCC nanoparticles have the desired properties necessary for serving as effective delivery vehicles for a wide variety of medicines, as well as for gene/protein delivery.77
Gelation from Polyelectrolyte Complex
This method involved ionotropic gelation, just as described in the preceding section, only in the present case the crosslinker was a polyanionic polymer with charges opposite to those of the chitosan derivative, with which it formed a PEC.
In a recent study, Mahjub et al conducted the preparation and optimization of N-trimethyl-O-carboxymethyl chitosan nanoparticles for the delivery of low-molecular-weight heparin. Nanoparticles were synthesized by the polyelectrolyte complexation technique. The optimization of nanoparticles was conducted using the Box-Behnken response surface experimental design methodology. The nanoparticles were characterized by their size, zeta potential, poly-dispersity index, entrapment efficiency, and loading efficiency, which were measured as 235 ± 24.3 nm, +18.6 ± 2.57 mV, 0.230 ± 0.03, 76.4 ± 5.43%, and 12.6 ± 1.37%, respectively. Examination of the morphology indicated the presence of individual spherical nanoparticles without any indication of clumping together. The in vitro release studies revealed that 93.6 ± 1.17% of enoxaparin was released from the nanoparticles after 600 minutes of incubation. The data gained has demonstrated that nanoparticles derived from trimethyl carboxymethyl chitosan are a promising option for the oral administration of enoxaparin.41
Chen et al examine the formation and properties of a novel polyelectrolyte complex of a drug carrier system for the delivery of doxorubicin (DOX), which consists of hyaluronic acid (HA) coated hydrophobically modified chitosan (CS). Various batches of different polyelectrolyte complexes by mixing deoxycholic acid (DCA) and chitosan (CS) in different amounts, namely 0.1, 0.2, and 0.3. These complexes were named CS-DCA10, CS-DCA20, and CS-DCA30, respectively. The particle sizes of the manufactured polyelectrolyte complex nanoparticles (PCNs) were determined to be within the range of 280–310 nm, which is bigger compared to the particle sizes of uncoated nanoparticles, which are approximately 150 nm. The PCNs have significant zeta potentials at approximately 26 mV, which contribute to their stability. No alterations in size were observed. The incorporation of DOX into the PCNs was achieved with high encapsulation efficiency (56%), which resulted in a sustained release profile without any sudden burst effect when exposed to PBS (pH 7.4) at 37 °C.97
Polymer Drug-Complexes
Trapani et al developed a nanoparticulate system using Cyclodextrin (CD)-Chitosan (CS) with sulphobutylether-β-CD (SBE-β-CD). This system has an inner core rich in CD and an outer layer rich in CS, which can encapsulate both hydrophilic and hydrophobic medicines. Subsequently, they investigated the administration of the glutathione (GSH) peptide through oral means using this nanoparticulate system. They then compared the results with nanoparticles made solely of CS or with a combination of CS and α-CD nanoparticles. The X-ray photoelectron spectroscopy investigation revealed the formation of a stable interaction between the peptide and SBE-β-CD at the inner core of the nanoparticles. However, it is noteworthy that this complexation exhibited exceptional stability, as it did not exhibit any release of GSH under conditions that simulate stomach activity.78
Maestrelli et al created a novel drug nanocarrier composed of chitosan and cyclodextrin nanoparticles. The interaction with cyclodextrin allows for the dissolution and safeguarding of delicate medicines, while their incorporation into the chitosan framework is anticipated to enhance their uptake. The medications were complexed with cyclodextrin, which promoted their entrapment into the nanoparticles. This resulted in a significant increase in the final drug loading of the nanoparticles, up to 4 times for triclosan and 10 times for furosemide. The findings of this study indicate that the drug-cyclodextrin complex was effectively preserved within the nanoparticulate structure. An initial rapid release phase and a subsequent delayed release phase defined the release profile of these nanoparticles in vitro.79
Self-Assembly
Hecq et al developed chitosan derivative nanoparticles containing insulin for oral administration. Chitosan derivative-based nanoparticles containing insulin were created through self-assembly, utilizing electrostatic interactions between the negatively charged medication and the positively charged polymers. The amine groups in chitosan derivatives were substituted to various extents (33, 52, or 99%) by 2-hydroxypropyl-3 trimethyl ammonium groups. This modification resulted in the polymers being persistently positively charged, regardless of the pH. The stability of the nanoparticles is vital for this sophisticated drug delivery system due to the varying pH levels in the gastrointestinal tract and the significance of electrostatic interactions. Mucoadhesion is further enhanced by the presence of permanent positive charges. Conversely, the electric charges of chitosan molecules are contingent upon the pH level of the surrounding medium. As a result of incorporating quaternary ammonium groups, the solubility of the chitosan derivatives was enhanced. To further strengthen the nanoparticles and ensure their stability, sodium tripolyphosphate (TPP) was introduced into the systems to generate additional cross-links. The presence of TPP affected both the breakdown of the polymer matrix and the subsequent release kinetics. The drug release processes were discovered to be more intricate than mere diffusion under stable conditions, potentially involving ionic interactions and matrix disintegration as well. The most favorable formulation utilized a chitosan derivative with a substitution degree of 33%. This formulation was characterized by a Z-average of 142 ± 10 nm, a zeta potential of 29 ± 1 mV, and an encapsulation efficacy of 52 ± 3%. Notably, the release of insulin was sustained for over 210 minutes.80
Yan et al developed a novel type of Tat tagged and folate-modified N-succinyl-chitosan (Tat-Suc-FA) self-assembly nanoparticles, to provide a new vector for tumor gene therapy. The nanoparticles were synthesized by distributing a certain quantity of Tat-Suc-FA copolymer into a 0.1% acetic acid solution (V/V) and then subjecting it to sonication using a probe-type sonicator (Sigma Ultrasonic Processor, Sonix-600) with a power output of 12W. The sonication was prolonged for 2 minutes. The self-aggregated solution was filtered using a membrane with a pore size of 0.22 µm and then kept at room temperature. The study effectively synthesized and characterized new Tat-Suc-FA copolymers. Tat-Suc-FA polymers effectively formed condensed complexes with plasmid DNA, resulting in positively charged complexes with widths ranging from 54 to 106 nm. The cytotoxicity of Tat-Suc-FA polymers in K562 cells was much decreased compared to that of chitosan.81
Potential Toxicity of Chitosan Nanoparticles
Chitosan, a natural polymer, is known for its biodegradability, biocompatibility, cost-effectiveness, and nontoxic properties. Its widespread use is notable in various countries including Japan, Italy, and Finland. In the United States, chitosan is classified as Generally Recognized as Safe (GRAS) by the Food and Drug Administration (FDA).82 Although the FDA has approved chitosan polymer for use in various healthcare products such as hemostatic dressings, contact lens coatings, and bandages, there are limited reports on its recognition and application as a drug delivery system.83 Several studies have reported the toxicity of chitosan, chitosan derivatives, and chitosan nanoparticles, but the toxicity of chitosan nanoparticle derivatives has not been widely reported. The toxicity of chitosan nanoparticles is generally influenced by the characteristics of the particles and cell lines
Particles Characteristic
The toxicity of chitosan nanoparticles, influenced by their characteristic, can be observed in vivo using various test animals such as rats, rabbits, dogs, and zebrafish. The selection of zebrafish is considered more advantageous because it does not require expensive costs. Additionally, zebrafish shares genomic similarities with humans, making it a test animal for assessing chitosan toxicity. Furthermore, zebrafish has a chorion with pore sizes ranging from 0.5 to 0.7 µm, allowing chitosan nanoparticles to enter through passive diffusion.84
Modification of chitosan in the form of nanoparticles can influence changes in the distribution profile and pharmacokinetics of the nanoparticles. This is influenced by the size and surface properties, where the positive charge of chitosan affects surface interactions that have implications on the kinetic profile of cellular absorption and chitosan nanoparticle toxicity.85 This positive charge also induces chitosan aggregation and red blood cell aggregation, leading to coagulation. This aggregation is considered toxic because when platelet aggregation occurs, it can result in thrombus formation and trigger thrombosis.86 Furthermore, Svirshchevskaya et al reported that chitosan and positively charged chitosan derivatives (Hydrophobizated and Quaternized Chitosan), when injected into a mouse at high concentrations (100 mg/Kg), caused embolism in small blood vessels and triggered death within 5–10 minutes post-injection. Additionally, chitosan and hydrophobic derivatives of chitosan were localized on the cell membrane. Quaternized chitosan was localized and found inside the cells, while the negatively charged chitosan derivative (N-Succinyl Chitosan) penetrated rapidly into the cells and did not activate platelet aggregation, making it assessed as safer, especially for Human Embryonic Kidney (HEK293) cells.87
However, this toxic property is influenced by several factors, including size, dosage, degree of deacetylation, and molecular weight. Hu et al reported that chitosan with a moderate degree of deacetylation (68.36%) had a faster coagulation time compared to chitosan with high deacetylation (92.21%) and low deacetylation (52.68%). The amine groups in chitosan in the right amount trigger stronger interactions with blood cells, while a higher degree of deacetylation is associated with more amine and hydroxyl groups in chitosan molecules, leading to the formation of a crystalline structure due to hydrogen bonding, thereby reducing the potential interaction between chitosan and blood cells.88
Modification of chitosan with a medium molecular weight can enhance the immune response in zebrafish but reduces the survival and swimming ability of embryos.89 Additionally, the dissolution of chitosan in acidic HCl and ddH2O media with higher pH shows differences in toxicity profiles. In acidic media, no significant toxicity is observed, while exposure to ddH2O media for 0.5 hours on zebrafish larvae causes damage to the yolk, considered an indicator of toxicity.90 In an in vivo study by Hu et al using zebrafish, it was observed that particles sized 340 nm at concentrations of 20 mg/L and 40 mg/L led to a considerable reduction in hatching, whereas concentrations of 40 mg/L of nanoparticles (340 nm) resulted in significant mortality of zebrafish embryos. Exposure to 200 nm particles at concentrations of 30 mg/L and 40 mg/L resulted in similar effects.91 Another study by Wang et al showed contrasting results, where chitosan concentrations of 200 mg/L, even with smaller particles of 84–86 nm, did not cause significant mortality.92 Additionally, in a study by Shaleh et al, chitosan nanoparticles ranging from 100 to 150 nm at concentrations of 200 mg/L demonstrated no toxic effects in zebrafish embryos, although higher concentrations led to a decrease in liver size, suggesting the potential for toxicity.93
Cell Lines
The observation of chitosan toxicity on tumor cells also indicates that chitosan exhibits lower toxicity compared to chitosan nanoparticles with a size of 40 nm, with an IC50 value of 15 µg/mL being more toxic than chitosan derivatives (20–2500 µg/mL).94 Additionally, this toxicity can be measured using mitochondrial dehydrogenase enzyme activity in cellular observations, particularly in Bipotential Human Liver (BHAL) cells. It is known that chitosan and chitosan nanoparticles at low concentrations, specifically 0.005% (w/v), show no signs of toxicity after 24 hours of incubation at physiological pH 6.0. However, at higher concentrations of 0.5%, with a 24-hour incubation period at pH 6.0, chitosan is found to have significantly higher enzyme activity compared to chitosan nanoparticles. This illustrates that the incubation time also influences chitosan toxicity, while the toxicity of chitosan nanoparticles can be influenced by the concentration of exposure.95
Toxicity testing of chitosan derivative in the form of pyridoxal chitosan nanoparticles did not show any potential toxicity in the MTT assay. This toxicity assessment was conducted by examining the cell viability percentage of the best nanoparticle formula at concentrations of 10, 300, and 3000 ppm, resulting in viability percentages of 98, 93, and 64%, respectively.96
Conclusion
Chitosan derivatives exhibit significant potential in the development of nanoparticle drug delivery systems. Future investigations should focus on the standardization of physicochemical properties of chitosan derivatives such as molecular weight, deacetylation degree, degree of substitution, and solubility since the various results from many studies reported. The derivative chitosan nanoparticles preparation using various methods to obtain the optimum method to produce chitosan derivative nanoparticles regarding the alteration of physicochemical properties of chitosan derivatives compared to native chitosan needs to be explored. The application of chitosan derivative-based nanoparticles in various fields needs to be conducted. The toxicity of chitosan derivative-based nanoparticles also needs to be explored. The pharmacokinetic profile which is related to the pharmacology effect of chitosan derivative-based nanoparticles and clinical trial should be conducted. The stability of chitosan-based nanoparticles needs to be considered due to the issue of agglomeration and environmental factors. By addressing these limitations through future research, chitosan derivative-based nanoparticles will gain more attention and more prospects to be applied in the pharmaceutical field.
Acknowledgments
The authors would like to thank the Rector of Universitas Padjadjaran, via the Directorate of Research and Community Engagement for APC funding.
Disclosure
The authors report no conflicts of interest in this work.
References
1. Tiyaboonchai W. Chitosan nanoparticles: a promising system for drug delivery. Naresuan Univ J. 2003;11:51–66.
2. Wang W, Chen S, Zhang L, et al. Poly(lactic acid)/chitosan hybrid nanoparticles or controlled release of anticancer drug. Mater Sci Eng C. 2015;46:514–520. doi:10.1016/j.msec.2014.10.048
3. Saheb M, Fereydouni N, Nemati S, et al. Chitosan-based delivery systems for curcumin: a review of pharmacodynamic and pharmacokinetic aspects. J Cell Physiol. 2019’2019:1–16.
4. Rashki S, Asgarpour K, Tarrahimofrad H, et al. Chitosan-based nanoparticles against bacterial infections. Carbohydr Polym. 2020;251:1–12.
5. Chen MC, Mi FL, Liao ZX, et al. Recent advances in chitosan-based nanoparticles for oral delivery of macromolecules. Adv Drug Deliv Rev. 2013;65:865–879. doi:10.1016/j.addr.2012.10.010
6. Trapani A, Garcia-Fuentes M, Alonso MJ. Novel drug nanocarriers combining hydrophilic cyclodextrins and chitosan. Nanotechnology. 2008;1:185101.
7. Sogias IA, Khutoryanskiy V, Williams AC. Exploring the factors affecting the solubility of chitosan in water. Macromol Chem Phys. 2010;211(4):426–433. doi:10.1002/macp.200900385
8. Gyu-yoon C, Korean Intellectual Property Office., assignee. Chitosan. Chitosan ester derivatives and methods for preparing the same (Chitosan Ester derivatives and method for preparation of the same). South Korea Patent KR20110111197A; 2010.
9. Suryani S, Chaerunisaa AY, Joni IM, et al. Production of low molecular weight chitosan using a combination of weak acid and ultrasonication methods. Polymers. 2022;14:1–15. doi:10.3390/polym14163417
10. Wang W, Meng Q, Li Q, et al. Chitosan derivatives and their application in biomedicine. Int J Mol Sci. 2020;21:1–26.
11. Mendoza JL, Monal WMA, Valencia FMG. Chemical Characteristics and Functional Properties of Chitosan. Cambridge Massachussets: Academic Press; 2016:3–31.
12. Zhao D, Yu S, Sun B, et al. Biomedical applications of chitosan and its derivative nanoparticles. Polymers. 2018;10(4):1–10. doi:10.3390/polym10040462
13. Bashir S, Teo YY, Ramesh S, et al. N-succinyl chitosan preparation, characterization, properties, and biomedical applications: a state of the art review. Rev Chem Eng. 2015;31:563–597.
14. Sashiwa H, Kawasaki N, Nakayama A, et al. Chemical modification of Chitosan. Part 15: synthesis of novel Chitosan derivatives by substitution of hydrophilic amine using N-carboxyethylchitosan ethyl ester as an intermediate. Carbohydr Res. 2003;338:557–561. doi:10.1016/S0008-6215(02)00492-5
15. Hirano S, Yamaguchi Y, Kamiya M. Water-soluble N-(n-Fatty acyl)chitosans. Macromol Biosci. 2003;3:629–631. doi:10.1002/mabi.200350029
16. Gonzalez PA, Justo JAZ, Lopez AS, et al. Gold nanoparticles with chitosan, N-acylated chitosan, and chitosan oligosaccharide as DNA carriers. Nanoscale Res Lett. 2019;258:1–14.
17. Yang Z, Peng H, Wang W, et al. Preparation, characterization, and protein loading properties of N-acyl chitosan nanoparticles. J Appl Polym Sci. 2010;16:1365–1371.
18. Cho Y, Kim JT, Park HJ. Size-controlled self-aggregated N-acyl chitosan nanoparticles as a vitamin C carrier. Carbohydr Polym. 2012;88:1087–1092. doi:10.1016/j.carbpol.2012.01.074
19. Prajapti C, Agrawal YO, Agnihotri VV, et al. Development and biological evaluation of protective effect of kidney targeted N - Acetylated Chitosan nanoparticles containing thymoquinone or the treatment of DNA damage in cyclophosphamide- induced haemorrhagic cystitis. Int J Biol Macromol. 2022;214:391–401. doi:10.1016/j.ijbiomac.2022.06.070
20. Park YS, Park HJ, Lee J. Stabilization of Glabridin by chitosan nano-complex. J Korean Soc Appl Biol Chem. 2012;5:457–462.
21. Naberezhnykh GA, Gorbach VI, Likhatskaya GN, et al. Interaction of N-acylated and N-alkylated chitosans included in liposomes with lipopolysaccharide of gram-negative bacteria. Biochem. 2013;78:301–308.
22. Chen Q, Qi Y, Jiang Y, et al. Progress in research of chitosan chemical modification technologies and their applications. Mar Drugs. 2022;20:1–36. doi:10.3390/md20080536
23. Pokhrel S, Yadav PN. Functionalization of Chitosan polymer and their applications. J Macromol Sci Part a Pure Appl Chem. 2019;56:450–475. doi:10.1080/10601325.2019.1581576
24. Ma G, Yang D, Zhou Y, et al. J preparation and characterization of water-soluble N -alkylated chitosan. Carbohydr Polym. 2008;74:121–126.
25. Yang TC, Chou CC, Li CF. Preparation, water solubility and rheological property of the N-alkylated mono or disaccharide chitosan derivatives. Food Res Int. 2002;35:707–713. doi:10.1016/S0963-9969(02)00064-9
26. Palacio DA, Urbano BF, Palencia M, et al. Preparation of alkylated chitosan-based polyelectrolyte hydrogels: the effect of monomer charge on polymerization. Eur Polym J. 2019;118:551–560. doi:10.1016/j.eurpolymj.2019.06.024
27. Chen H, Cui S, Zhao Y, et al. O-alkylation of chitosan for gene delivery by using ionic liquid in an in- situ reactor. Engineering. 2012;04(10):114–117. doi:10.4236/eng.2012.410B029
28. Robles E, Villar E, Alatorre-Meda M, et al. Effects of the hydrophobization on chitosan-insulin nanoparticles obtained by an alkylation reaction on Chitosan. J Appl Polym Sci. 2013;129:822–834. doi:10.1002/app.38870
29. Liu C, Wang J, Huang S, et al. Self-assembled nanoparticles for cellular delivery of peptide nucleic acid using amphiphilic N, N, N-trimethyl-O-alkyl chitosan derivatives. J Mater Sci Mater Med. 2018;29:1–14. doi:10.1007/s10856-018-6120-y
30. Pinto RJB, Fernandes SCM, Freire CSR, et al. Antibacterial activity of optically transparent nanocomposite films based on chitosan or its derivatives and silver nanoparticles. Carbohydr Res. 2012;348:77–83. doi:10.1016/j.carres.2011.11.009
31. Jimtaisong A, Saewan N. Utilization of carboxymethyl chitosan in cosmetics. Int J Cosmet Sci. 2014;36:12–21. doi:10.1111/ics.12102
32. Boamah P, Huang Y, Hua M, et al. Sorption of heavy metal ions into carboxylate chitosan derivatives-a mini-review. Ecotoxicol Environ Saf. 2015;116:113–120. doi:10.1016/j.ecoenv.2015.01.012
33. Song Q, Zhang Z, Gao J, et al. Synthesis and property studies of N-carboxymethyl chitosan. J Appl Polym Sci. 2010;116:2658–2667.
34. Mohamed NA, Mohamed RR, Seoudi RS. Synthesis and characterization of some novel antimicrobial thiosemicarbazone O-carboxymethyl chitosan derivatives. Int J Biol Macromol. 2014;63:63–69. doi:10.1016/j.ijbiomac.2013.10.044
35. Patale RL, Patravale VB. O, N-carboxymethyl chitosan-zinc complex: a novel chitosan complex with enhanced antimicrobial activity. Carbohydr Polym. 2011;85:105–110. doi:10.1016/j.carbpol.2011.02.001
36. Manimohan M, Pugalmani S, Sithique MA. Biologically active water soluble novel biopolymer/hydrazide based O-carboxymethyl chitosan Schiff bases: synthesis and characterisation. J Inorg Organomet Polym Mater. 2020;30:3658–3676. doi:10.1007/s10904-020-01487-9
37. Wang H, Gong X, Miao Y, et al. Preparation and characterization of multilayer films composed of chitosan, sodium alginate and carboxymethyl Chitosan-Zno nanoparticles. Food Chem. 2019;283:397–403. doi:10.1016/j.foodchem.2019.01.022
38. Huang YC, Kuo TH. O-carboxymethyl Chitosan/fucoidan nanoparticles increase cellular curcumin uptake. Food Hydrocoll. 2016;53:261–269. doi:10.1016/j.foodhyd.2015.02.006
39. Liu S, Yang S, Ho PC. Intranasal administration of carbamazepine-loaded carboxymethyl chitosan nanoparticles for drug delivery to the brain. Asian J Pharm Sci. 2018;13:72–81. doi:10.1016/j.ajps.2017.09.001
40. Feng C, Wang Z, Jiang C, et al. Chitosan/O-carboxymethyl chitosan nanoparticles for efficient and safe oral anticancer drug delivery: in vitro and in vivo evaluation. Int J Pharm. 2013;457:158–167. doi:10.1016/j.ijpharm.2013.07.079
41. Mahjub RL, Heidari ST, Radmehr M, et al. Preparation and optimization of N-trimethyl-O-carboxymethyl chitosan nanoparticles for delivery of low-molecular-weight heparin. Pharm Dev Technol. 2016;21:14–25. doi:10.3109/10837450.2014.965320
42. Chaiwarit T, Sommano SRP, Kantrong N, et al. Development of carboxymethyl chitosan nanoparticles prepared by ultrasound-assisted technique for a clindamycin HCl carrier. Polymers. 2022:14. doi:10.3390/polym15010014
43. Xu C, Xing R, Liu S, et al. The immunostimulatory effects of hydroxypropyltrimethyl ammonium chloride chitosan-carboxymethyl chitosan nanoparticles. Int J Biol Macromol. 2021;181:398–409. doi:10.1016/j.ijbiomac.2021.03.148
44. Pedro RDO, Schmitt CC, Neumann MG. Syntheses and characterization of amphiphilic quaternary ammonium chitosan derivatives. Carbohydr Polym. 2016;147:97–103. doi:10.1016/j.carbpol.2016.03.083
45. Andreica B, Cheng X, Marin L. Quaternary ammonium salts of Chitosan. A critical overview of the synthesis and properties generated by quaternization. Eur Polym J. 2020;139:1–16. doi:10.1016/j.eurpolymj.2020.110016
46. Fan L, Yang J, Wu H, et al. Preparation and characterization of quaternary ammonium chitosan hydrogel with significant antibacterial activity. Int J Biol Macromol. 2015;79:830–836. doi:10.1016/j.ijbiomac.2015.04.013
47. Sajomsang W, Gonil P, Tantayanon S. Antibacterial activity of quaternary ammonium chitosan containing mono or disaccharide moieties: preparation and characterization. Int J Biol Macromol. 2009;44:419–427. doi:10.1016/j.ijbiomac.2009.03.003
48. Naskar S, Koutsu K, Sharma S. Chitosan-based nanoparticles s drug delivery systems: a review n two decades of research. J Drug Target. 2019;27:379–393. doi:10.1080/1061186X.2018.1512112
49. Mi F-L, Wu -Y-Y, Lin Y-H, et al. Oral delivery of peptide drugs using nanoparticles self-assembled by poly(Γ-glutamic acid) and A chitosan derivative functionalized by trimethylation. Bioconjug Chem. 2008;19(6):1248–1255. doi:10.1021/bc800076n
50. Li SD, Li PW, Yang ZM, et al. Synthesis and characterization of chitosan quaternary ammonium salt and its application as drug carrier for ribavirin. Drug Deliv. 2014;21:548–552. doi:10.3109/10717544.2013.853708
51. Ahmed TA, Aljaeid BM. Preparation, characterization, and potential application of chitosan, chitosan derivatives, and chitosan metal nanoparticles in pharmaceutical drug delivery. Drug Des Devel Ther. 2016;10:483–507. doi:10.2147/DDDT.S99651
52. Hu F, Zhou Z, Xu Q, et al. Novel pH-responsive quaternary ammonium chitosan-liposome nanoparticles for periodontal treatment. Int J Biol Macromol. 2019;129:1113–1119. doi:10.1016/j.ijbiomac.2018.09.057
53. Huang TW, Ho YC, Tsai TN, et al. Enhancement of the permeability and activities of epigallocatechin gallate y quaternary ammonium chitosan/fucoidan nanoparticles. Carbohydr Poly. 2020;242:1–9. doi:10.1016/j.carbpol.2020.116312
54. Wang J, Wang H. Preparation of soluble P-aminobenzoyl chitosan ester by schiff’s base and antibacterial activity of the derivatives. Int J Biol Macromo. 2011;48:523–529. doi:10.1016/j.ijbiomac.2011.01.016
55. Jayakumar R, Nwe N, Tokura S, et al. Sulfated chitin and chitosan as novel biomaterials. Int J Biol Macromol. 2007;40(3):175–181. doi:10.1016/j.ijbiomac.2006.06.021
56. Li Y, Chen XG, Liu N, et al. Physicochemical characterization and antibacterial property of chitosan acetates. Carbohydr Polym. 2007;67:227–232. doi:10.1016/j.carbpol.2006.05.022
57. Luangtana-anan M, Nunthanid J, Limmatvapirat S. Potential of different salt forming agents on the formation of chitosan nanoparticles as carriers for protein drug delivery systems. J Pharm Investi. 2019;49:37–44. doi:10.1007/s40005-017-0369-x
58. Al RZ, Abulateefeh SR, Taha MO. Synthesis and characterization of chitosan-lactate – phthalate and evaluation of the corresponding zinc- and aluminum- crosslinked beads as potential controlled release matrices. Eur Polym. 2015;73:402–412. doi:10.1016/j.eurpolymj.2015.11.004
59. Bhattari N. Preparation of lactid acid-grafted chitosan nanoparticles as carriers for prolonged drug delivery. Int J Nanomed. 2006;1:181–187. doi:10.2147/nano.2006.1.2.181
60. Jadidi-Niaragh F, Atyabi F, Rastegari A, et al. CD73 specific siRNA loaded chitosan lactate nanoparticles potentiate the antitumor effect of a dendritic cell vaccine in 4T1 breast cancer bearing mice. J Control Release. 2017;246:46–59. doi:10.1016/j.jconrel.2016.12.012
61. Colonna C, Conti B, Perugini P, et al. Chitosan glutamate nanoparticles for protein delivery: development and effect on prolidase stability. J Microencapsul. 2007;24:553–564. doi:10.1080/02652040701449608
62. Sandhya M, Aparna V, Maneesha KS, et al. Amphotericin B loaded sulfonated chitosan nanoparticles for targeting macrophages to treat intracellular candida glabrata infections. Int J Biol Macromol. 2018;110:133–139. doi:10.1016/j.ijbiomac.2018.01.028
63. Ding N. Homogeneous etherification modification of chitosan and preparation of high-strength hydrogel. J Phys Conf Ser. 2022;2261:1–8. doi:10.1088/1742-6596/2261/1/012011
64. Wang J, Zhuang S. Chitosan-based materials: preparation, modification and application. J Clean Prod. 2022;355:1–10. doi:10.1016/j.jclepro.2022.131825
65. Cao J, You J, Zhang L, et al. Homogeneous synthesis and characterization of chitosan ethers prepared in aqueous alkali/urea solutions. Carbohydr Polym. 2018;185:138–144. doi:10.1016/j.carbpol.2018.01.010
66. Putra P, Husni A, Puspita ID. Characterization and application of N, O-carboxy methyl chitosan produced at different temperature of etherification. Int J Pharm Clin Res. 2016;8:1493–1498.
67. Zou X, Zhao X, Ye L. Synthesis of cationic chitosan hydrogel with long chain alkyl and its controlled glucose-responsive drug delivery behavior. RSC Adv. 2015;5:96230–96241. doi:10.1039/C5RA16328E
68. Yang R, Li H, Huang M, et al. A review on chitosan-based flocculants and their applications in water treatment. Water Res. 2016;95:59–89. doi:10.1016/j.watres.2016.02.068
69. Sun M, Wang T, Pang J, et al. Hydroxybutyl chitosan centered biocomposites for potential curative applications: a critical review. Biomacromolecules. 2020;21(4):1351–1367. doi:10.1021/acs.biomac.0c00071
70. Xie W. Preparation and antibacterial activity of a water-soluble chitosan derivative. Carbohydr Polym. 2002;50(1):35–40. doi:10.1016/S0144-8617(01)00370-8
71. Sun M, Yu X, Wang T, et al. Nasal adaptive chitosan-based nano-vehicles for anti-allergic drug delivery. Int J Biol Macromol. 2019;135:1182–1192. doi:10.1016/j.ijbiomac.2019.05.188
72. Lu YH, Liu ZM, Cheng DH. Preparation of hydroxypropyl chitosan nanoparticles and their application in Antheraea pernyi silk treatment. Adv Mater Res. 2013;796:380–384. doi:10.4028/www.scientific.net/AMR.796.380
73. Hoang NH, Thanh TL, Sangpueak R, et al. Chitosan nanoparticles-based ionic gelation method: a promising candidate for plant disease management. Polymers. 2022;14(4):1–28. doi:10.3390/polym14040662
74. Algharib SA, Dawood A, Zhou K, et al. Preparation of chitosan nanoparticles by ionotropic gelation technique: effects of formulation parameters and in vitro characterization. J Mol Struct. 2022;1252:67–78. doi:10.1016/j.molstruc.2021.132129
75. Abrica-González P, Zamora-Justo JA, Sotelo-López A, et al. Gold nanoparticles with chitosan, N-acylated chitosan, and chitosan oligosaccharide as DNA carriers. Nanoscale Res Lett. 2019;14(1):258. doi:10.1186/s11671-019-3083-y
76. Canepa C, Imperiale JC, Berini CA, et al. Development of drug delivery SYSTEM based on chitosan nanoparticles for oral administrations of interferon-alpha. Bio Macromolecules. 2017;18:3302–3309. doi:10.1021/acs.biomac.7b00959
77. Sayın B, Somavarapu S, Li XW, et al. Mono-N-Carboxymethyl Chitosan (MCC) and N-Trimethyl Chitosan (TMC) nanoparticles for non-invasive vaccine delivery. Int j Pharm. 2008;363:139–148.
78. Maestrelli F, Garcia-Fuentes M, Mura P, et al. A new drug nanocarrier consisting of chitosan and hydoxypropylcyclodextrin. Eur J Pharm Biopharm. 2006;63:79–86. doi:10.1016/j.ejpb.2005.12.006
79. Hecq J, Siepmann F, Siepmann J, et al. Development and evaluation of chitosan and chitosan derivative nanoparticles containing insulin for oral administration. Drug Dev Indust Pharm. 2015;41(12):2037–2044. doi:10.3109/03639045.2015.1044904
80. Yan CY, Gu JW, Hou DP, et al. Synthesis of tat tagged and folate modified N-succinyl-chitosan self-assembly nanoparticles as a novel gene vector. Int J Bio Macromol. 2015;72:751–756. doi:10.1016/j.ijbiomac.2014.09.031
81. Rostami E. Progresses in targeted drug delivery system using chitosan nanoparticles in cancer therapy: mini review. J Drug Delivery Sci Technol. 2020;20:1–28.
82. Shahbazi Y, Shavisi N. Current advancements in application of chitosan based nano metal oxide as food preservative materials. Nanomed Res Journal. 2019;4:122–129.
83. Fako VE, Furgeson DY. Zebrafish as a corelative and predictive model for assessing biomaterial nanotoxicity. Adv. Drug Delivery Rev. 2020;61:478–486. doi:10.1016/j.addr.2009.03.008
84. Dou T, Wang J, Han C, et al. Cellular uptake and transport characteristics of chitosan modified nanoparticles in Caco-2 cell monolayers. Int J Biol Macromol. 2019;138:791–799. doi:10.1016/j.ijbiomac.2019.07.168
85. Zoe LH, David SR, Rajabalaya R. Chitosan nanoparticles toxicity: a comprehensive literature review of in vivo and in vitro assessments for medical applications. Toxicol Rep. 2023;11:83–106. doi:10.1016/j.toxrep.2023.06.012
86. Svirshchevskaya EV, Zubareva AA, Boyko AA, et al. Analysis of toxicity and biocompatibility of chitosan derivatives with different physico-chemical properties. Appl Biochem Microbiol. 2016;52:483–490. doi:10.1134/S000368381605015X
87. Hu Z, Cheng SLY, Kong S, et al. Investigation of the effect of molecular parameters on the hemostatic properties of chitosan. Molecules. 2018;23:1–14. doi:10.3390/molecules23123147
88. Nikapitiya C, Dananjaya SHS, Silva BCJD, et al. Chitosan nanoparticles: a positive immune response modulator as displays in zebrafish larvae against Aeromonas hydrophila infection. Fish Shellfish Immunol. 2018;76:240–246. doi:10.1016/j.fsi.2018.03.010
89. Chou CM, Mi FL, Horng JL, et al. Characterization and toxicology evaluation of low molecular weight chitosan on zebrafish. Carbohydr Polym. 2020;240:1–12. doi:10.1016/j.carbpol.2020.116164
90. Hu YL, Qi W, Han F, et al. Toxicity evaluation of biodegradable chitosan nanoparticles using a zebrafish embryo model. Int j Nanomed. 2011;6:3351–3359. doi:10.2147/IJN.S25853
91. Wang Y, Zhou J, Liu L, et al. Characterization and toxicology evaluation of chitosan nanoparticles on the embryonic development of zebrafish, Danio rerio. Carbohydr. Polym. 2016;141:204–210. doi:10.1016/j.carbpol.2016.01.012
92. Abou-Saleh H, Younes N, Rasool K, et al. Impaired liver size and compromised neurobehavioral activity are elicited by chitosan nanoparticles in the zebrafish embryo model. Nanomaterials. 2019;9(1):1–13. doi:10.3390/nano9010122
93. Qi L, Xu Z, Jiang X, et al. Cytotoxic activity of chitosan nanoparticles and copper loaded nanoparticles. Bioorg. Med. Chem. Lett. 2005;15:1397–1399. doi:10.1016/j.bmcl.2005.01.010
94. Loh JW, Yeoh G, Saunders M, et al. Uptake and cytotoxicity of chitosan nanoparticles in human liver cells. Toxicol Appl Pharmacol. 2010;249(2):148–157. doi:10.1016/j.taap.2010.08.029
95. Kritchenkov AS, Kurasova MN, Godzishevskaya AA, et al. High antibacterial activity and low toxicity of pyridoxal derivatives of chitosan and their nanoparticles. Mendeleev Commun. 2021;31(4):504–506. doi:10.1016/j.mencom.2021.07.022
96. Jafernik K, Ladniak A, Blicharska E, et al. Chitosan-based nanoparticles as effective drug delivery systems—a review. Molecules. 2023;28(4):1–17. doi:10.3390/molecules28041963
97. Chen L, Zheng Y, Feng L. Novel hyaluronic acid coated hydrophobically modified chitosan polyelectrolyte complex for the delivery of doxorubicin. Int J Biol Macromol. 2018;12:215.
© 2024 The Author(s). This work is published and licensed by Dove Medical Press Limited. The full terms of this license are available at https://www.dovepress.com/terms.php and incorporate the Creative Commons Attribution - Non Commercial (unported, v3.0) License.
By accessing the work you hereby accept the Terms. Non-commercial uses of the work are permitted without any further permission from Dove Medical Press Limited, provided the work is properly attributed. For permission for commercial use of this work, please see paragraphs 4.2 and 5 of our Terms.