Back to Journals » Breast Cancer: Targets and Therapy » Volume 15
Evaluation of Cytotoxicity of the Methanolic Extract of Red Sea Marine Sponge Xestospongia Testudinaria and Its Related Compounds Against MCF-7 Human Breast Cancer Cells
Authors Alkhilaiwi FA , Fadil SA , Aljoud FA, Yonbawi AR, Ashi A , Hareeri RH, Bakhashab S , Alamri AM, Albikairi AY, Shaala LA , El-Gamal AA, Youssef DT
Received 9 July 2023
Accepted for publication 28 November 2023
Published 7 December 2023 Volume 2023:15 Pages 879—890
DOI https://doi.org/10.2147/BCTT.S429721
Checked for plagiarism Yes
Review by Single anonymous peer review
Peer reviewer comments 2
Editor who approved publication: Professor Pranela Rameshwar
Faris A Alkhilaiwi,1,2 Sana A Fadil,1,2 Fadwa A Aljoud,2,3 Ahmed R Yonbawi,1 Abrar Ashi,2,4 Rawan H Hareeri,5 Sherin Bakhashab,6 Ahmad M Alamri,7,8 Ahmed Y Albikairi,1 Lamiaa A Shaala,9,10 Ali A El-Gamal,11,12 Diaa T Youssef1,10,13
1Department of Natural Products and Alternative Medicine, Faculty of Pharmacy, King Abdulaziz University, Jeddah, 21589, Saudi Arabia; 2Regenerative Medicine Unit, King Fahd Medical Research Center, King Abdulaziz University, Jeddah, 21589, Saudi Arabia; 3Scientific Research Center, Dar Al-Hekma University, Jeddah, 22246, Saudi Arabia; 4Department of Medical Laboratory Sciences, Faculty of Applied Medical Sciences, King Abdulaziz University, Jeddah, Saudi Arabia; 5Department of Pharmacology and Toxicology, Faculty of Pharmacy, King Abdulaziz University, Jeddah, 21589, Saudi Arabia; 6Department of Biochemistry, Faculty of Science, King Abdulaziz University, Jeddah, 21589, Saudi Arabia; 7Department of Clinical Laboratory Sciences, College of Applied Medical Sciences, King Khalid University, Abha, 61481, Saudi Arabia; 8Cancer Research Unit, King Khalid University, Abha, 61481, Saudi Arabia; 9Suez Canal University Hospital, Suez Canal University, Ismailia, 41522, Egypt; 10Natural Products Unit, King Fahad Medical Research Center, King Abdulaziz University, Jeddah, 21589, Saudi Arabia; 11Department of Pharmacognosy, College of Pharmacy, King Saud University, Riyadh, 11451, Saudi Arabia; 12Department of Pharmacognosy, Faculty of Pharmacy, Mansoura University, El-Mansoura, 35516, Egypt; 13Department of Pharmacognosy, Faculty of Pharmacy, Suez Canal University, Ismailia, 41523, Egypt
Correspondence: Faris A Alkhilaiwi; Diaa T Youssef, Email [email protected]; [email protected]
Background: Breast cancer is a leading cause of death and one of the most common fatal medical conditions in the world. Chemical compounds of various types have been identified in the Red Sea marine sponge Xestospongia testudinaria, including sterol esters, sterols, indole alkaloids, and brominated polyunsaturated fatty acids. These compounds have demonstrated promising biological features, which in cludes anti-inflammatory, cancer preventive, and antioxidant capacities.
Methods: The cytotoxic potential of Xestospongia testudinaria was assessed by the 3-(4,5-dimethylthiazol-2-yl)-2,5-diphenyltetrazolium bromide (MTT) assay and morphological alterations in MCF-7 cell line. Furthermore, the flow cytometry was also utilized to assess apoptosis and identify changes in the cell cycle; besides, cell migration was assessed by scratch wound-healing assay.
Results: A significant dose-dependent decrease in the percentage of MCF-7 cell viability was observed with IC50 39.8 ug/mL. Functional studies were performed on MCF-7 to show that Xestospongia testudinaria raises apoptotic cell death and induces growth arrest at the G1/G0 while inhibiting cell migration in scratch assay.
Conclusion: These results demonstrated that Xestospongia testudinaria extract has an inhibitory effect on breast cancer cells proliferation, migration and induce apoptosis. Thus, it holds great promise as a potential treatment for breast cancer.
Keywords: Red Sea sponge, Xestospongia testudinaria, breast cancer cell lines, cytotoxic activity
Graphical Abstract:
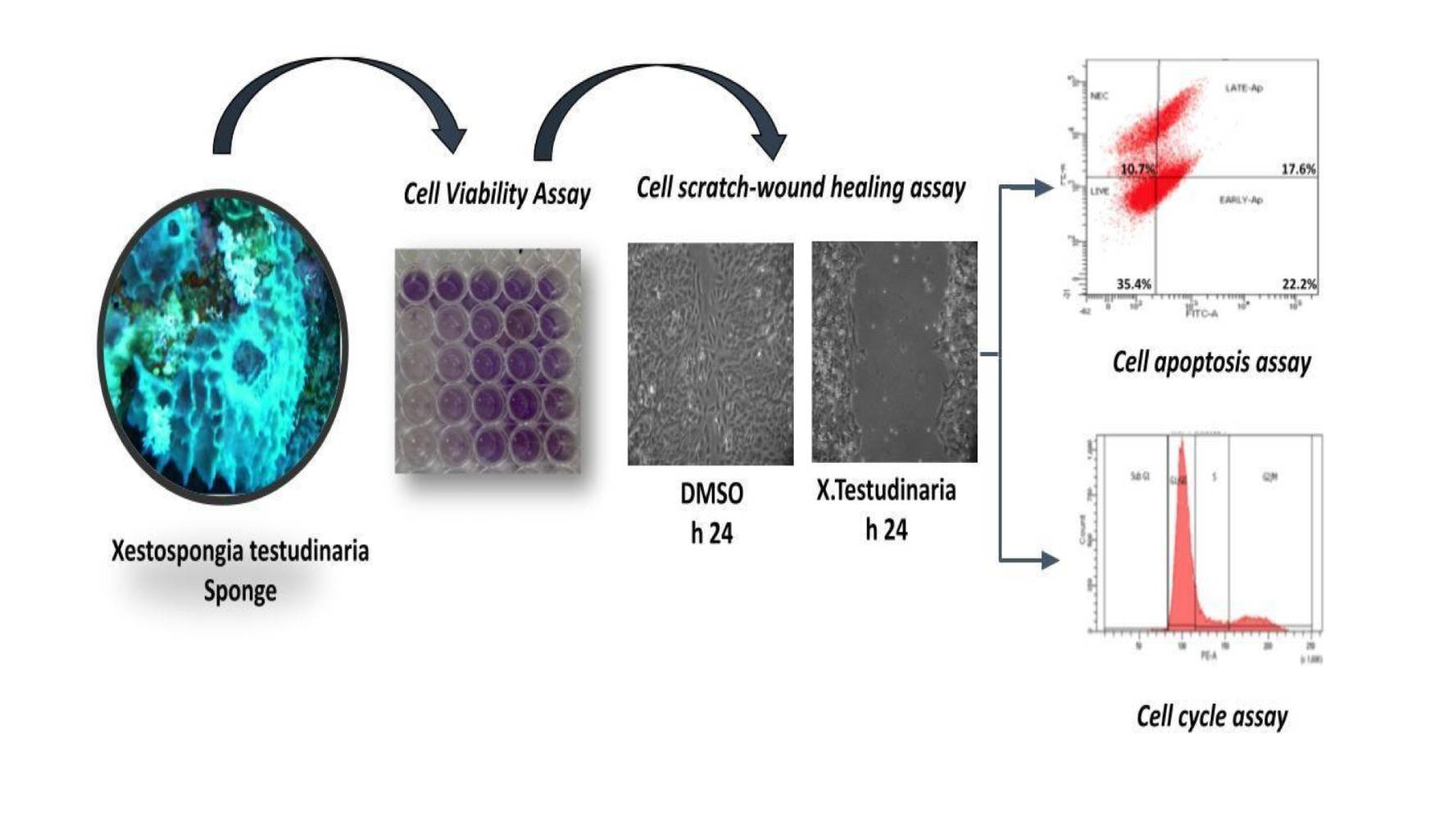
Introduction
Cancer is the second fastest-growing cause of death in approximately 185 countries, accounting for over 10 million deaths.1 It is anticipated that the incidence of cancer will persistently increase in the forthcoming decades as a result of shifts in population demographics. By the year 2040, it is projected that there will be approximately 16.3 million cancer-related deaths and an additional 27.5 million new cases of cancer worldwide.2,3 The diagnosis rate for breast cancer is the highest among all types of cancer in Saudi Arabia.2,3 Chemotherapy is currently commonly used in conjunction with surgery, radiation, and other cancer treatments, though these procedures have not always resulted in the best results.4,5 The development of innovative anticancer drugs has gained importance because the currently available cancer medications are expensive and have limited selectivity.6 In search for novel anticancer chemicals, a wide variety of natural resources, including marine organisms, have been considered.
Marine sponges (phylum Porifera) have been reported to be an abundant source of biologically active substances with various biological properties, making them crucial lead compounds for drug development.7 These organisms are classified as one of the most primitive and ancient species found in various oceanic environments. They possess exceptional resilience in the face of challenging conditions, including low temperatures, high pressure, varying salinity levels, and predatory threats. Sponge defence mechanisms have evolved over thousands of years by producing secondary metabolites that aid in their defence against harmful bacteria, algae, fungi, and other potential predators.6 The research of the sponge Xestospongia Testudinaria secondary metabolites (family Petrosiidae), frequently referred to as barrel sponges has been repeated in many parts of the world since the 1970s when marine natural compounds research began.8 These secondary metabolites contain a variety of chemical substances such as isoquinoline, quinones, sterols, brominated polyacetylenic acids, macrocyclic quinolizidines, pyridoacridine alkaloids, and esters.9 We focused on the Red Sea species, Xestospongia testudinaria because previous studies had shown the remarkable bioactivities of the crude extract, which included antimicrobial, cytotoxic, anti-inflammatory, vasodilatation, immunomodulatory, antioxidant, inhibition of HIV protease, cardiotonic stimulation, insecticidal activity, and antiplasmodial activity.9–12 In keeping with the ongoing focus in finding possible marine-derived bioactive chemicals for treating modern illnesses like cancer, the Red Sea sponge Xestospongia testudinaria’s cytotoxic potential was carefully examined. Our team discovered complete alcoholic extracts and n-hexane fraction of the extracts of the sponge X. testudinaria were cytotoxic to human liver cancer cell line (HepG-2), metastatic cervical cancer (HeLa), and human medulloblastoma-derived cell line (Daoy).13 The objective of this study was to investigate the potential anticancer properties of the methanol-based extract derived from the Red Sea Marine Sponge X. testudinaria. Specifically, the study aimed to assess the extract’s ability to inhibit the proliferation and migration of the MCF-7 cell line, a malignant strain associated with breast cancer. The findings of this research could provide valuable insights into the potential therapeutic application of the extract for breast cancer treatment.
Materials and Methods
Sponge Material
The SCUBA divers collected the sponge by hand from Abulat Island off Al-Lith (N019o56’31.6”, E040o08’17.3”) in the Red Sea, Saudi Arabia, at a depth of between 17 and 21 metres. After collection, the sponge was immediately kept on ice and then frozen until it was processed. Professor Rob van Soest of the Naturalis Biodiversity Center in the Netherlands determined that the sponge was a specimen of the Xestospongia testudinaria genus. One of the samples of the sponge was kept in the Red Sea Marine Invertebrates Collection at King Abdulaziz University, and it had the number DY-12 associated with it, while another sample was kept in the Naturalis Biodiversity Center’s collections under the collection number RMNH Por. 9176. The sponge’s detailed description had already been published.9
Extraction and Isolation
To extract the sponge’s biological compounds, the researchers freeze-dried it and crushed the lyophilized material (52 g). They then extracted it three times at room temperature with a mixture of MeOH-CH2Cl2 (1:1) totalling 300 mL. After vacuum evaporation, the combined extracts were concentrated for further testing in biological systems.
Cell Viability Assay
The effect of the organic extract of X. testudinaria or a single compound on cell viability of MCF-7 was evaluated by MTT assay (Solarbio, Beijing, China). Briefly, MCF-7 cells (3x103 cells/well) were plated overnight in a 96-well plate to ensure adhesion, then the cells were exposed to the extract of X. testudinaria (7.8-15.5-31.25-62.5-125 ng/μL) or purified compounds (labelled 1–15) (3, 6, 12, 25, 50 µM) and 0.5% DMSO as a vehicle control, for 48h. For 3T3 cells, complete DMEM media were used for culturing and 3×103 cells/well was the density of cells as well. After incubating at 37°C for 4 hours, 100 µL of the 5 mg/mL MTT solution was added to every single well. The supernatant was then discarded, and 100 μL of DMSO was added to each well and incubated there for 10 minutes at 37 °C. Absorbance at 490 nm was measured using a microplate reader (BioTek, Winooski, VT, USA), and OD values was used to calculated cell viability (%). Using the GraphPad 9.0 software (GraphPad Software Inc., California, United States), we were able to calculate the half-maximal inhibitory concentration (IC50). MCF-7 cells were acquired from the American Type Culture Collection (ATCC,USA) and 3T3 cells from kerafast, USA.
Cell Apoptosis Assay
Following the manufacturer’s instructions, the PI/Hoechst 33342 apoptosis detection kit (Solarbio, China) was used to measure apoptosis, and a FACSAria III flow cytometer was used to collect 10,000 events (BD, Bioscience, San Jose, CA, USA). The MCF-7 cells were treated with IC50 concentration (39.8 ug/mL) for 48h before performing apoptosis assay. Apoptotic percentage was calculated by summing of early (Hoechst+PI−) and late apoptotic (Hoechst+PI+) cells.
Cell Cycle Assay
Following a treatment of X. testudinaria extract on MCF-7 cells for a period of 48 h, the cells were washed with phosphate-buffered saline (PBS). After that, the cells were fixed using 70% pre-cold ethanol overnight, washed with PBS and then incubated with 100 μL RNase A for 30 min at 37°C. Subsequently, 400 μL propidium iodide (PI) were added to the cells and incubated for 30 min in the dark at 4°C. All procedures were conducted according to the protocol from the DNA Content Quantitation kit (Solarbio, Beijing, China) by acquiring 10,000 events using FACSAria III flow cytometer.
Cell Scratch-Wound Healing Assay
Each well of the six-well plates was seeded with approximately 25×103 MCF-7 cells, and they were given the night to adhere. Mitomycin C (5 g/mL; Roche, Germany) was applied to the cells for two hours to prevent them from proliferating. A scratch was drawn vertically at the center of the well and then the wounded cells’ monolayer was photographed at 0h, 24h and 48 h in both groups. Wound healing gap was measured using image J (LOCI, University of Wisconsin).
Evaluation of Mitochondrial Membrane Potential (JC-1 Assay)
After trypsinization, the cells were counted prior to dilution with serum-containing culture medium (DMEM, 10% heat-inactivated FBS, 1% antibiotics, and 1% L-glutamine). Cells were seeded at a density of 1.5×105 cells per mL, and 1 mL was added to a 24-well plate. The plate was then incubated for 24 hours at 37 °C, with 5% CO2 and 95% relative humidity. After the incubation period, the supernatant was carefully aspirated, and treatment at IC50 concentration was applied; each experiment included wells for negative and positive controls.
Mediums were removed from wells and washed twice with PBS before adding 500 µL serum-free culture medium and 500 µL of JC-1 dyeing working solution to each well, then incubated for 20 min at 37 °C. JC-1 accumulates within fully functional mitochondria and generates J-aggregates (red colour), and the colour of the dye shifts from red to green if the mitochondrial membrane potential is depolarized. The supernatant was discarded and then washed twice with a JC-1 dyeing buffer. Finally, 1 mL of serum-free culture medium was added to each well before obtaining images with a Leica fluorescence microscope. The captured images were processed using ImageJ.
Reverse Transcriptase and Quantitative PCR
Following the manufacturer’s instructions, total RNA was extracted using the miRNeasy Mini kit (catalogue number 217004) purchased from Qiagen in Hilden, Germany. The NanoDropTM spectrophotometer was used to measure the RNA’s concentration and purity. The QuantiTect Reverse Transcription Kit with the catalogue number 205311 from Qiagen in Hilden, Germany, was utilised for the synthesis of cDNA. qPCR reactions were performed using Real-time PCR SYBR Green 1 kit (Cat no. SR1110, Solarbio, China). Each PCR reaction was carried out in triplicates in a 96-well plate using qPCR thermocycler for 40 cycles (Applied Biosystem). Relative amounts of cDNA were normalised to the internal control GAPDH. The RT-qPCR data was analysed to obtain the relative gene expression of the targeted genes and the corresponding controls in each experiment using the 2−ΔΔCt method. Primers for MMP2, MMP9 and housekeeping gene GAPDH (macrogen) primers are MMP2 Forward 5’-CCGTCGCCCATCATCAAGTTC-3’ Reverse 5’-GCAGCCATAGAAGGTGTTCAGG-3’ MMP9 Forward 5’-TGGTCCTGGTGCTCCTGGTG-3’ Reverse 5’-GCTGCCTGTCGGTGAGATTGG-3’ GAPDH Forward 5’-TTCCTACCCCCAATGTATCCG-3’ Reverse 5’-CCACCCTGTTGCTGTAGCCATA-3’.
Statistical Analysis
The data are presented as the mean with standard deviation (SD). In statistical analyses, the differences between control (DMSO) and organic extract of X. testudinaria were compared using the Student’s t-test. The GraphPad Prism 9.0 software (GraphPad, San Diego, CA, USA) was used to perform the calculations. A p-value of less than 0.05 was considered to be the threshold for a statistically significant result. Statistical significance is indicated as * P<0.05, ** P<0.01, *** P<0.001 **** P<0.0001. There was a total of three separate experiments conducted for each possible outcome.
Results
The Effect of X. testudinaria Extract on MCF-7 Cell Morphology and Cell Proliferation
Phase contrast microscopy was employed to observe the MCF-7 cells after exposure to either dimethyl sulfoxide (DMSO) or various concentrations of the extract derived from X. testudinaria. The cells were cultured for a duration of 24 hours. The results indicated a notable reduction in cell numbers when compared to the control group. The observed cellular manifestations included stress-induced phenotypes such as cellular shrinkage and disruptions in the cell membrane, ultimately resulting in cell death (Figure 1A). The MCF-7 cells were treated with the extract of X. testudinaria at various concentrations (7.8-15.5-31.25-62.5-125 ng/μL) for 48 h demonstrated concentration dependent on decrease in cell proliferation (Figure 1B, left panel). The IC50 for the time point 48 h was calculated as 39.8 ug/mL. Moreover, the extract of X. testudinaria showed no significant inhibitory effect on normal cells 3T3 even at the highest concentration (Figure 1B, right panel).
Extract of X. testudinaria Increases Apoptosis and G1/G0 Cell Cycle Arrest in MCF-7 Cell Line
To elucidate the mechanism by which the extract of X. testudinaria enhances cytotoxicity in MCF-7 cell line, apoptosis and cell cycle analysis were assessed by flow cytometry. A viable dose of 39.8 ug/mL of extract corresponding to IC50 for MCF-7 cells was selected for the assays. Figure 2A and B depict the results of the experiment, demonstrating that treatment with the specified concentration of extract led to a significant increase in MCF-7 apoptosis (3.7-fold, p = 0.0002) after 48 hours of treatment, as compared to the control group. Furthermore, the treatment with the extract resulted in a substantial augmentation of G1/G0 cell cycle arrest in the MCF-7 cells, as depicted in Figure 3A and B.
Extract of X. testudinaria Inhibits MCF-7 Migration
MCF-7 cells were treated with 39.8 ug/mL of the extract from X. testudinaria reduced cell migration at 24h (p < 0.001) and 48h (p < 0.001, Figure 4A and B) compared to controlled sample. Moreover, RT-PCR results showed significant decrease in MMP-2 and MMP-9 mRNA expression levels (P<0.0001 and P<0.05 respectively, Figure 4C) in MCF-7 cells due to the effect of X. testudinaria.
The Effect of X. testudinaria on Mitochondrial Membrane Potential
The potential mitochondrial membrane potential was evaluated using a fluorescence microscope and the JC-1 staining technique to validate cytotoxicity data. Cells were stained with JC-1 dye that aggregates within active mitochondria and emits red fluoresces. Cells treated with X. testudinaria exhibited a significant decrease in red/green fluorescence intensity compared to the negative control (p < 0.01). As observed from the image, X. testudinaria induced depolarization of the mitochondrial membrane with emitted green fluorescence (Figure 5A and B).
The Effect of the Pure Compounds Isolated from the Extract of X. testudinaria on MCF-7 Cell Survival
The cells MCF-7 were treated, separately, with 11 out of 15 compounds purified previously from the sponge X. testudinaria.13 To assess the impact of individual compounds (C) on cell survival, a range of concentrations (3, 6, 12, 25, 50 µM) were employed to treat the cells for a duration of 48 hours. There was no effect on cell survival of MCF-7 on all studied concentrations of the 11 compounds (Figure 6). Compounds number 5, 6, 8 and 12 (structures are not shown) were not tested due to the lack of enough materials (Table 1).
![]() |
Table 1 Structures and Names of Tested Compounds |
![]() |
Figure 6 The cytotoxicity of MCF-7 after treatments of various concentrations of individual compounds obtained from X. testudinaria for 48 hours. Data from three replicates shown as mean ± SD (n=3). |
Discussion
Breast Cancer, a highly significant global health concern, has garnered substantial research interest aimed at revealing its complicated characteristics and identifying novel molecules for efficacious therapeutic interventions. Numerous drug discovery initiatives are currently prioritizing the investigation of natural products that possess abundant molecular scaffolds known for their successful utilization in the development of anti-cancer agents.14 Marine sponges, classified under the Phylum Porifera, are recognized as the most ancient multicellular organisms and represent a highly valuable reservoir of pharmaceutical prospects.15 Despite their sessile lifestyle and typically soft bodies, marine sponges excel at producing cytotoxic secondary metabolites. This allows them to gain an advantage in the competitive struggle for space on the limited substrate of a reef by slowing the growth of neighboring organisms through reduced cell division.16 Therefore, in the case of sponges, the cytotoxicity of the compounds can serve as a reliable indicator of their potential to proliferate in coral habitats and subsequently hinder the growth of cancer cells. These characteristic holds promise for the development of novel drugs.17 The process of developing anticancer drugs commences with the evaluation of the cytotoxicity of screening compounds against various cancer cell lines. This approach is considered optimal for treating cancer, as the objective is to selectively eliminate tumour cells while preserving the viability of normal cells.18 The genus Xestospongia (family Petrosiidae) is represented in the Red Sea and have numerous secondary metabolites such as isoquinoline, sterols, brominated polyacetylenic acids, macrocyclic quinolizidines, pyridoacridine alkaloids, quinones, and esters.8
Our group has found that the combined effect of alcohol and n-hexane X. testudinaria extracts exhibited significant cytotoxic effects on various cancer cell lines, including human cervical tumors (HeLa), human liver cancer (HepG-2), and human medulloblastoma (Daoy).13 In this particular study, our focus was on examining the bioactivity of the organic extract derived from the Red Sea sponge X. testudinaria. We specifically aimed to assess its impact on the MCF-7 breast cancer cell line, with a particular emphasis on its anti-proliferation effects. Additionally, we sought to identify the specific compound within X. testudinaria that is responsible for its efficacy by isolating and investigating a total of 11 compounds.
The extract derived from X. testudinaria exhibits a significant impact on both the morphology and proliferation of MCF-7 cells when compared to cells that have not been subjected to any treatment. The proliferation of the MCF-7 cell line exhibited a gradual decrease from day 0 to day 2, with an observed IC50 value of 39.8 ug/mL. This indicates the biological cytotoxic effect on cell proliferation which agrees with a previous study done on Hella cell line using X. testudinaria extract.19 Furthermore, it was observed that the extracts derived from X. testudinaria exhibit a significant capacity to induce apoptosis in MCF-7 cells that were subjected to treatment, as compared to the cells that were not treated. The aforementioned discovery indicates the potential role of X. testudinaria extract in modulating the apoptosis pathway.
Furthermore, the administration of X. testudinaria extract resulted in a notable augmentation of G1/G0 cell cycle arrest in MCF-7 cells that were subjected to treatment, as compared to cells that were not treated. Previous studies have established that cellular response to gamma irradiation or chemotherapeutic agents results in the induction of G1/G0 phase arrest. This regulatory process is mediated by the p53 protein and ultimately results in apoptosis.20,21 Our finding suggested that the extracts of X. testudinaria has a critical role in controlling G1/G0 cell cycle arrest.
Cell migration is considered a hallmark of cancer metastasis.22 The migration analysis study elucidated inhibition of treated MCF-7 cells migration significantly compared to control during different endpoint times. Matrix metalloproteinases 2 (MMP-2) and (MMP-9) degrade the extracellular matrix, which has a major impact on cell invasion and migration in both pathological and physiological conditions.23,24 Additionally, MDA-MB-231 and MCF-7 cells and breast cancer tissue samples showed significantly higher levels of MMP-2 and MMP-9 when compared to normal tissues and normal cells.18,19 It is clear from our study that X. testudinaria extract regulates the cell migration pathway by decreasing the expression level of MMP-2 and MMP-9 which are involved in the migration process.
Mitochondrial membrane potential in MCF-7 cells after treatment with X. testudinaria was analysed using the fluorescent dye JC-1, which aggregates into healthy mitochondria. Red fluorescence is diminished because JC-1 dye is redistributed throughout the cell rather than accumulating at the mitochondria during the mitochondrial collapse in apoptotic cells. We confirmed this by observing a reduction in the intensity of the red fluorescence, which indicates that the mitochondrial membrane potential was disturbed by the X. testudinaria extract.
Pure pharmaceutical compounds that are manufactured on an industrial scale or extracted from botanical sources are often selected based on their potent efficacy against specific diseases in humans.24 Nevertheless, the level of activity exhibited by the isolated compounds from X. testudinaria at proportional concentrations is significantly lower compared to that of the unrefined extract. The occurrence of positive interactions among various components within the whole plant extracts may provide an explanation for the observed phenomenon of crude extracts being more efficacious than isolated constituents when administered at comparable doses. There is often scientific proof of synergistic effects, although the precise underlying mechanisms remain to be fully understood. The literature has extensively documented the existence of synergistic effects among various constituents of extracts, demonstrating their efficacy not only in anti-cancer activity but also in other pharmacological activities.25 The efficacy of the X. testudinaria extract surpasses that of an isolated compound when administered at an equivalent dosage. Further investigation is necessary in order to validate if there is of synergy, enhanced bioavailability, cumulative effects, or additive properties.
Conclusions
In conclusion, X. testudinaria extract shows potential inhibition in cancer cell line growth and migration capability. To date, no other report has reported cytotoxicity of X. testudinaria extract to the MCF-7 cells. A future direction for this study should be to investigate more bioactivity involvement of X. testudinaria extract in the critical cancer pathogenesis and signalling pathway. Additionally, it is imperative to conduct additional research in order to examine the potential of combining pure compounds in clinical trials, with the aim of further evaluating the effectiveness and safety of the X. testudinaria extract.
Acknowledgments
AcknowledgmentsThis research work was funded by the Institutional Funds Projects under grant no (IFPRC-003-290-2020). Therefore, the authors gratefully acknowledge the technical and financial support from the Ministry of Education and King Abdulaziz University, Jeddah, Saudi Arabia.
Author Contributions
All authors made substantial contributions to conception and design, acquisition of data, or analysis and interpretation of data; took part in drafting the article or revising it critically for important intellectual content; agreed to submit to the current journal; gave final approval of the version to be published; and agree to be accountable for all aspects of the work.
Funding
This research was funded by the Institutional Fund Projects (grant no. IFPRC-003-290-2020).
Disclosure
The authors declare that they have no conflicts of interest in this work.
References
1. Sung H, Ferlay J, Siegel RL, et al. Global Cancer Statistics 2020: GLOBOCAN Estimates of Incidence and Mortality Worldwide for 36 Cancers in 185 Countries. CA Cancer J Clin. 2021;71(3)209–249. doi:10.3322/caac.21660
2. World Health Statistic. Monitoring Health for the SDGs. World Health Statistic; 2019.
3. Almutlaq BA, Almuazzi RF, Almuhayfir AA, et al. Breast cancer in Saudi Arabia and its possible risk factors. J Cancer Policy. 2017;12. doi:10.1016/j.jcpo.2017.03.004
4. Urruticoechea A, Alemany R, Balart J, Villanueva A, Vinals F, Capella G. Recent advances in cancer therapy: an overview. Curr Pharm Des. 2009;16(1):3–10.
5. Baskar R, Lee KA, Yeo R, Yeoh KW. Cancer and radiation therapy: current advances and future directions. Int J Med Sci. 2012;9:193–199. doi:10.7150/ijms.3635
6. Maeda H, Khatami M. Analyses of repeated failures in cancer therapy for solid tumors: poor tumor‐selective drug delivery, low therapeutic efficacy and unsustainable costs. Clin Transl Med. 2018;7(1). doi:10.1186/s40169-018-0185-6
7. Rinehart KL. Secondary metabolites from marine organisms. Ciba Found Symp. 1992;171:236–49; discussion 249–54. doi:10.1002/9780470514344.ch14
8. Zhou X, Xu T, Yang XW, et al. Chemical and biological aspects of marine sponges of the genus Xestospongia. Chem Biodivers. 2010;7:2201–2227. doi:10.1002/cbdv.201000024
9. El-Shitany NA, Shaala LA, Abbas AT, et al. Evaluation of the anti-inflammatory, antioxidant and immunomodulatory effects of the organic extract of the red sea marine sponge xestospongia testudinaria against carrageenan induced rat paw inflammation. PLoS One. 2015;10(9). doi:10.1371/journal.pone.0138917
10. Chaidir LWH, Ebel R, Edrada RA, et al. Rocaglamides, glycosides, and putrescine bisamides from Aglaia dasyclada. J Nat Prod. 2001;64(9):1216–1220. doi:10.1021/np0102354
11. Ying Z, Xu S, Wei C, et al. Epidemiological investigation on a cluster epidemic of COVID-19 in a collective workplace in Tianjin. Chin J Endemiol. 2020;41(5):648–652.
12. Patil AD, Kokke WC, Cochran S, Francis TA, Tomszek T, Westley JW. Brominated polyacetylenic acids from the marine sponge xestospongia muta: inhibitors of HIV protease. J Nat Prod. 1992;55(9):1170–1177. doi:10.1021/np50087a002
13. El-Gamal AA, Al-Massarani SM, Shaala LA, et al. Cytotoxic compounds from the Saudi Red Sea Sponge Xestospongia testudinaria. Mar Drugs. 2016;14:82. doi:10.3390/md14050082
14. Harvey AL, Edrada-Ebel R, Quinn RJ. The re-emergence of natural products for drug discovery in the genomics era. Nat Rev Drug Discov. 2015;14(2):111–129. doi:10.1038/nrd4510
15. Hentschel U, Piel J, Degnan SM, Taylor MW. Genomic insights into the marine sponge microbiome. Nat Rev Microbiol. 2012;10(9):641–654. doi:10.1038/nrmicro2839
16. Aerts LAM. Sponge/coral interactions in Caribbean reefs: analysis of overgrowth patterns in relation to species identity and cover. Mar Ecol Prog Ser. 1998;175. doi:10.3354/meps175241
17. Lee OO, Wang Y, Yang J, Lafi FF, Al-Suwailem A, Qian PY. Pyrosequencing reveals highly diverse and species-specific microbial communities in sponges from the Red Sea. ISME J. 2011;5(4):650–664. doi:10.1038/ismej.2010.165
18. Aslantürk ÖS. In Vitro Cytotoxicity and Cell Viability Assays: principles, Advantages, and Disadvantages. In: Genotoxicity - A Predictable Risk to Our Actual World; 2018. doi10.5772/intechopen.71923
19. Swantara MD, Rita WS, Suartha N, Agustina KK. Anticancer activities of toxic isolate of Xestospongia testudinaria sponge. Vet World. 2019;12(9):1434–1440. doi:10.14202/vetworld.2019.1434-1440
20. Levine AJ. p53, the cellular gatekeeper for growth and division. Cell. 1997;88(3):53. doi:10.1016/S0092-8674(00)81871-1
21. Geurts JMW, Schoenmakers EFPM, Röijer E, Aström AK, Stenman G, van de Ven WJM. Identification of NFIB as recurrent translocation partner gene of HMGIC in pleomorphic adenomas. Oncogene. 1998;16(7):865–872. doi:10.1038/sj.onc.1201609
22. Entschladen F, Palm D, Drell T, Lang K, Zaenker K. Connecting a tumor to the environment. Curr Pharm Des. 2007;13(33):3440–3444. doi:10.2174/138161207782360573
23. Li H, Qiu Z, Li F, Wang C. The relationship between MMP-2 and MMP-9 expression levels with breast cancer incidence and prognosis. Oncol Letters. 2017. doi:10.3892/ol.2017.6924
24. Wagner H, Ulrich-Merzenich G. Synergy research: approaching a new generation of phytopharmaceuticals. Phytomedicine. 2009;16:97–110. doi:10.1016/j.phymed.2008.12.018
25. Williamson EM. Synergy and other interactions in phytomedicines. Phytomedicine. 2001;8(5). doi:10.1078/0944-7113-00060
© 2023 The Author(s). This work is published and licensed by Dove Medical Press Limited. The full terms of this license are available at https://www.dovepress.com/terms.php and incorporate the Creative Commons Attribution - Non Commercial (unported, v3.0) License.
By accessing the work you hereby accept the Terms. Non-commercial uses of the work are permitted without any further permission from Dove Medical Press Limited, provided the work is properly attributed. For permission for commercial use of this work, please see paragraphs 4.2 and 5 of our Terms.